1. INTRODUCTION
Wood is a renewable and versatile material that is highly sought after in the 21st century. The increasing demand for wood products can be reflected in the recent growth of the global forest area that was once on a rapid decline and is projected to grow to 4.1 billion hectares by 2030 (Ghani and Lee, 2021). This trend positively impacts timber industries of countries like Malaysia as wood is the third most important commodity along with oil palm and rubber for international trades and exports for the country. In 2020, Malaysia boasted a total estimate timber export value of RM 22 billion. In 2021, despite the COVID-19 pandemic, the export value increased to RM 22.74 billion. The country’s lucrative and established timber industry can be attributed to the diverse forestry which covers 50% of the country (Prime Minister's Office of Malaysia, 2023) and demand for wood products by countries such as the U.S., Japan, and Europe (Malaysia Timber Industry Board, 2021; University of Cambridge, 2021).
A major contributor to the timber industry is the dipterocarpeaece family particularly the Shorea sp. or known locally as meranti. Meranti is native to Southeast Asia and can be commonly found in natural tropical forests in Peninsular Malaysia. It is part of a family of 535 species and 17 genera of Dipterocarps. Amongst this family, meranti is highly sought after due to its colours, strength, pleasant appearance, and workability. It’s commonly used for doors, window frames, decking, furniture, paneling, and light construction applications (Tay, 2023). This timber species is heavily exported especially to places such as Europe for doors and windows construction due to their availability and technical properties (Pablopublishing, 2019).
The year-on-year increase in demand for high quality timber products put tremendous strain on natural, or primary forests in Malaysia. From 2002 to 2022, the country has lost 2.85 Mha or 18% of humid primary forest largely due to logging activities. Governing bodies have initiated various countermeasures such as stricter law enforcement, the ecological fiscal transfer for biodiversity conservation initiatives, and the pledge to maintain 50% of forest land coverage in the country at the 1992 Rio Earth Summit to reduce primary forest loss (Global Forest Watch, 2023). Aside from increasing longevity of the forests, increasing the service life of timber-products is an important consideration especially through natural means. This is because timber or wood is highly susceptible to biodegradation if left untreated (Belt et al., 2024).
Throughout the years, wood preservatives such as those with arsenic and chromium content have been banned due to their harm caused to the environment and the users (Baharuddin et al., 2022; Ibáñez et al., 2023). A potential candidate that solves this issue are waterborne wood preservatives, particularly boron compounds (Augustina et al., 2020; Priadi et al., 2020). Boron compounds are not toxic to humans, and environmentally friendly which are essential for wooden products (Zhang et al., 2023). Boron compounds have been considered as good alternatives for preserving wood from various threats such as insects, fungus, and bacterial attack (Ng et al., 2023). Boron compounds are commonly used in our daily lives in items such as laundry detergents, cosmetics, plant food, and eye drops. It is an excellent replacement for conventional wood preservative as it is no more acutely toxic than table salt (Bhatia, 2002; Wilson and Yost, 2000). Yamaguchi (2003, 2005) highlighted that boron compounds are environmentally acceptable wood preservative alternatives. The problem with boron compounds is their inherent low solubility into wood as highlighted by (Nunes et al., 2023). Factors such as the wood type, sample size, preservative concentration, and preservative treatment type often vary from studies as they contribute significantly to the efficacy of the treatment such as in Colakoglu et al. (2003), Kurt (2008), Yusof et al. (2023).
Treatability variability amongst meranti species is not well documented with many preservatives. There is a lack of knowledge and systematic approach to effectively enhance the physical and mechanical properties of hard wood species like meranti with different preservative methods. Pande et al. (2007) concluded that to secure even small absorption of preservatives, drastic schedules were required for impregnating the timber, meranti pa’ang group and meranti dammar hitam group. Ani et al. (2005) estimated that the service life different meranti groups in ground-contact is around 1.1 to 3.6 years and highlights the non-durable characteristics of meranti in outdoor applications. Light red, white, and yellow meranti are non-durable in contract with ground or in exposed conditions whereas dark red meranti is moderately durable. Salamah et al. (1991) highlighted that dipping yellow, white, and light red meranti (LRM) into Bis (tributyltin) oxide, TBTO is suitable for protection of timber against insect and fungi after processing (molding and drilling).
According to some studies, wood treated with boron compounds, especially boric acid (BA) tends to negatively impact the mechanical properties of wood (Colakoglu et al., 2003). However, another study showed that BA treatment enhances properties such as the surface roughness, modulus of elasticity (MOE), and density of wood (Simsek et al., 2010). A study by Taylor et al. (2023), concluded that vacuum treating CLT panels with borate provides sufficient levels of preservative retention and has minimal effects on mechanical properties. From the studies mentioned, the lack of systematic approaches to enhance the physical and mechanical properties of timber products limits boron compounds’ commercial viability.
This research project aims to quantify the influence of boron preservatives on the physical and mechanical properties of LRM using ASTM testing standards. As popular studies use boron variations such as copper chrome boron (Selamat et al., 1992) or DOT (Freeman et al., 2013), this research project focuses on BA to treat LRM. For the physical properties, two factors were taken, concentration of BA and sample sizes. For mechanical properties, only the concentration of BA was taken into consideration. Analysis of variance (ANOVA) tests were conducted to determine the statistical significance between the factors tested.
2. MATERIALS and METHODS
In this study, LRM or Shorea leprosula, which is frequently used in indoor applications and has a huge distribution market domestically and internationally, is used as the sample material. The LRM used in the preparation of samples was obtained from local timber industry in Miri, Sarawak. The wood samples obtained were without defects and paying close attention to the fiber direction of each sample. The wood samples were left to dry under room conditions until air-dried moisture content (MC) is achieved ~12%.
BA, which is a waterborne wood preservative was used in the impregnation process. The solution was prepared by dissolving varying concentrations of BA, namely 3%, 5%, and 7% with respect to weight quantity into separate plastic basins. The aqueous solution was prepared before fully submerging the wooden samples into the plastic basins. The commercial grade BA (H3BO3) was used in this study was the boron compound of choice for the impregnation process.
LRM samples were prepared in accordance with ASTM D1037 – 06a standards. This applies to all the physical and mechanical tests namely, retention and MC, and bending strength, apparent MOE, tensile and compression strength parallel against the grain respectively. For physical properties, the 20 samples were measured, 5 for each category of variable (0%, 3%, 5%, and 7% BA concentration). The same allocation of number of samples was applied to measuring the mechanical properties.
The samples used for measuring the physical properties were reused to measure the mechanical properties as the only data needed was weight. The wooden samples prepared were checked for defects, paying close attention to the fiber direction to ensure that no flaws were present. Samples found with flaws that were not in accordance with required standards were excluded for the test (Hadi et al., 2019). The aim is to minimize uncertainties due to defective test samples. The different wood samples are shown in Fig. 1.
The applied test standards, number of samples and dimensions for determining the physical properties of the wooden samples were given in Table 1. The applied test standards, number of samples and dimensions for determining the mechanical properties of the wooden samples were given in Table 2.
The method used was the dip diffusion method. The samples were labelled according to their respective tests. The preservative concentration ranged from 3%, 5%, to 7% aqueous BA solution which is higher than the average concentration used compared to other studies (Colakoglu et al., 2003; Tripathi, 2012; Yusof et al., 2023).
The samples were initially dried in a Memmert-UN30 Universal Oven at 103 ± 2°C for 24 hours to obtain oven dried weight (Submited and Salihu, 2014). The samples were then fully submerged into either one of the four different BA concentrations of plastic basins for 72 hours. A control was introduced with 0% BA concentration. After the soaking treatments, the samples were dried to oven-dried weight for another 24 hours at 103 ± 2°C. Throughout the process, the samples were measured with an electronic balance for their pre-treatment and post-treatment oven-dried weight while ensuring no moisture is present on the surface of samples. The physical properties such as retention rate and MC can be obtained by using the difference in weight between phases. Mechanical tests were carried out on the oven-dried impregnated and control samples. Fig. 2 shows the experimental setup of the post-submerged impregnated samples.
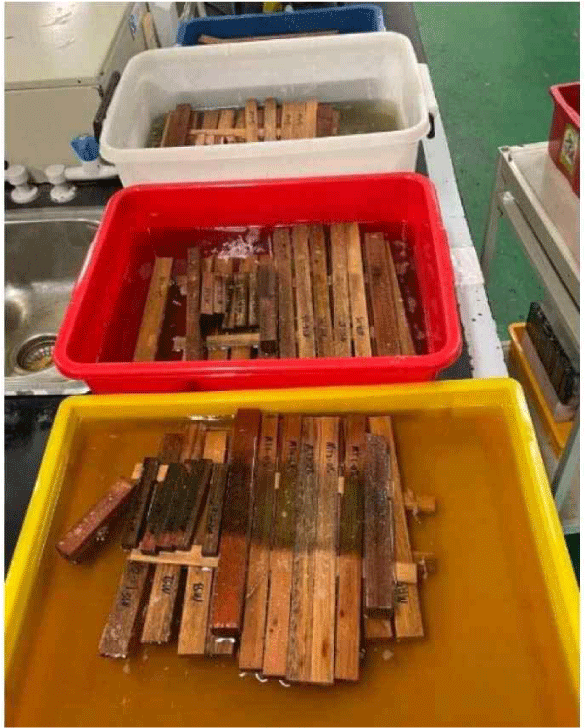
The physical properties determined in this study include retention rate and MC.
The retention rate, R of the impregnated wood samples was determined by conducting a volumetric analysis in accordance with ASTM 1413 (ASTM, 2007). The net uptake of BA was calculated by measuring the differences in weight between the pre-treatment. The formula to measure retention rate is shown in Equation (1) (Priadi et al., 2023):
Where, G is the difference between the weight of test samples before and after impregnation, g; C is the solution concentration, %; V is the volume of the test samples, cm3.
The MC of the impregnated and control samples under air dried conditions was determined in accordance with ASTM 4442 (ASTM, 1992). The MC was calculated by measuring the differerce between the pre-treatment oven-dried and air-dried weight. The formula to measure MC is shown in Equation (2) (Priadi et al., 2021):
Where, Mi is the air-dried weight of the sample, g; Mo is the oven-dried weight of the sample.
The mechanical properties determined in this study include bending strength, apparent MOE, tensile strength parallel to the grain, and compression strength parallel to the grain.
The bending strength, modulus of rupture (MOR), was determined in accordance with ASTM D 1037 – 06a (ASTM, 2006). The dimensions of test samples prepared were 260 × 25 × 15 mm in accordance with these standards. The length of the samples was set at about 17 times that of the thickness (t × 17 = 15 × 17 = 255 mm). The samples were loaded on a Universal Testing Machine at the midpoint marked on their surface with the load applied to the top surface of the specimen. A uniform loading rate was set to 3.8 mm/min. The load (Pmax) on the instant of breaking point was observed and the bending strength was calculated according to (3):
Where, Pmax is the load at the instant of breaking point, N; L is the length of the sample, mm; w is the width of the sample, mm; t is the thickness of the sample, mm.
The apparent MOE was determined in accordance with ASTM D 1037 – 06a (ASTM, 2006). The dimensions of test samples prepared were 260 × 25 × 15 mm in accordance with these standards. The length of the samples was set at about 17 times that of the thickness (t × 17 = 15 × 17 = 255 mm). The samples were loaded on a Universal Testing Machine at the midpoint marked on their surface with the load applied to the top surface of the specimen. A uniform loading rate was set to 3.8 mm/min. The setup for the wood samples used to calculate the bending strength was used to calculate the apparent MOE. The slope of the straight-line portion of the load-deflection curve (△P/△y) was observed and the apparent MOE was calculated according to Özcan and Korkmaz (2019):
Where, △P/△y is the slope of the straight-line portion of the load-deflection curve, N/mm; L is the length of the sample, mm; w is the width of the sample, mm; t is the thickness of the sample, mm.
The tensile strength parallel to the grain, Rt was determined in accordance with ASTM D 1037 – 06a (ASTM, 2006). The dimensions of test samples prepared were 300 × 20 × 4 mm in accordance with these standards. The samples were loaded on a Universal Testing Machine by clamping each end of the specimen with fastening grips. The total effective length of the sample is 210 mm excluding the 90 mm of wood that was used on the fastening grips at each end. The uniform loading rate was set to 4 mm/min. The load (Pmax) on the instant of breaking point was observed and the tensile strength parallel to the grain was calculated according to Park and Jo (2020):
Where, Pmax is the load at the instant of breaking point, N; w is the width of the sample, mm; t is the thickness of the sample, mm.
The compression strength parallel to the grain, Rc was determined in accordance with ASTM D 1037 – 06a (ASTM, 2006). The dimensions of test samples prepared were 110 × 15 × 10 mm in accordance with these standards. The samples were loaded on a Universal Testing Machine (Instron 5982, Instron, Norwood, MA, USA) by placing the samples securely in between the bearing plates. The uniform loading rate was set to 0.55 mm/min. The load (Pmax) on the instant of breaking point was observed and the compression strength parallel to the grain was calculated according to Augustina et al. (2023):
Where, Pmax is the load at the instant of breaking point, N; w is the width of the sample, mm; t is the thickness of the sample, mm.
An ANOVA test was carried out to determine whether the factors tested, namely BA concentration and sample sizes had significant interaction effects of 5% or lower. Two types of ANOVA tests were conducted namely one-way (mechanical properties) and two-way (physical properties). The ANOVA tests were calculated using the ANOVA: single factor function in excel for the one-way test and SPSS Version 27 software for Windows for the two-way test.
3. RESULTS and DISCUSSION
The results measured for the physical and mechanical properties for LRM treated with varying concentrations of BA were presented along with discussions on the data obtained.
An analysis of the different physical properties namely the retention rate, and MC of the samples of different dimensions and BA concentrations is given in average values in Table 3.
An ANOVA test from the statistical information on the determination of the physical properties highlighted is given in Table 4. Graphical representations of the results are shown in Fig. 3.
As shown in Table 3, MC for samples from all three tests is on average 13.05% with the minimum and maximum being 11.79% and 14.21% respectively. This shows that the MC of all the samples is in air-dried condition (~12%) before proceeding with the experiment (Fidan and Adanur, 2019). This is to ensure that the samples experience the same dimensional changes, and no defects are caused by excessive shrinkage when oven dried (Damayanti et al., 2020). It should be noted that the samples were oven-dried in an oven before the impregnation process to empty the internal spaces of wood for maximum retention and even distributed of solution across the sample (Bergman, 2021).
The highest retention rate of samples was 20.69 kg/m3 in samples of test number 2 with 7% BA solution and the lowest retention rate of samples was 5.37 kg/m3 in test 1 with 3% BA solution. It was found that the retention rate increased as the boron acid concentration increased in all tests that were carried out. This can be attributed to the fact that as boron concentration increases, the amount of boron substrate to bond with wood increases. Additionally, the recommended retention rate of BA is 8 kg/m3 to prevent insects such as termites and decay by fungi in tropical countries such as Malaysia or Indonesia (Larasati and Sulistyo, 2014). The samples all showed retention rates over 8 kg/m3 except for test 1 with 3% BA and test 3 with 3% BA which shows that the retention rate is sufficient to prevent insect attacks. The highest mean retention rate was found in samples of test 2 followed by test 3 then 1 as the surface-area-to-volume ratio, SA to Vol ratio is highest in samples of test 2 (SA to Vol ratio of tests 1, 2, and 3 are 0.27, 1.01, and 0.42, respectively). The results obtained are consistent with a study conducted by Toker (2007). In Toker’s study, he impregnated oriental beech woods with varying concentrations of borax and BA. The retention rates of 1%, 3%, and 5% BA and borax were 4.95 kg/m3 and 5.03 kg/m3, 13.86 kg/m3 and 15.20 kg/m3, 26.69 kg/m3 and 25.22 kg/m3, respectively. This study concluded that the retention rate and the boron compound concentration had a linear relationship with each other.
Aside from retention rate, analysis of the depth of penetration is important to ensure the quality of the treatment. A study by Tripathi (2012) showed that despite the lower retention rate of red meranti (2.9 kg/m3) compared to yellow meranti (4.5 kg/m3), the depth of penetration is greater with a value of 7.2 mm (red) compared to 5.0 mm (yellow). This study does not offer a test on the depth of penetration which means that the quality of BA treatment cannot be verified with just the results of retention rate. Additionally, steps should be taken to assess the depth of penetration along with its retention rate due to these two variables being mutually exclusive.
From Table 4, the statistical analysis of the data using the ANOVA test indicates that there are significant interaction effects at 5% level of significance between all factors (BA concentration, sample sizes, BA concentration × sample sizes) tested. The results indicate that the effects of sample size varied among the concentrations of the preservative and vice versa.
An analysis on the statistical information of the mean values for the mechanical properties was given Table 5 relating to the bending strength, apparent MOE, tensile strength parallel to fiber grains, and compression strength parallel to fiber grains. The results of the ANOVA test were presented in Table 6 to determine the significance of the findings. A graphical representation of the mechanical properties obtained is shown in Fig. 4.
The highest mean bending strength value, MOR in test 1 is 92.65 kPa with 7% BA concentration and the lowest value is 67.41 kPa with 3% BA concentration. The bending strength of the impregnated samples show a linearly increasing relationship as the bending strength increases from 67.42 kPA with 3% BA concentration to 92.65 with 7% BA concentration. The control samples with 0% BA concentration have a mean bending strength of 81.08 kPa which is higher than the bending strength of samples with 3% and 5% BA concentration. This can be attributed to the fact that during the impregnation process, the crystalline boron salt diffuses and is placed between the micelles in the cell wall, decreasing the bonding properties of the cellulosic material, wood (Young, 2007).
The highest MOE value in test 1 is 197.24 MPa with 7% BA concentration and the lowest value is 161.59 MPa with 5% BA concentration. The mean MOE values show a decrease from 3% to 5% BA concentration which is 177.50 MPa to 161.59 MPa. An increase in tensile strength then proceeded from 5% to 7% BA concentration which is 161.59 MPa to 197.24 MPa respectively. The control samples with 0% BA concentration have a mean MOE value of 121.69 MPa which is less than all the samples that were impregnated with BA.
The highest mean tensile strength parallel to fiber grains, Rt in test 2 is 49.98 MPa with 5% BA concentration and the lowest mean value is 44.78 MPa with 3% BA concentration. The mean tensile strength shows an increase from 3% to 5% BA concentration which is 44.78 MPa to 49.98 MPa. A decrease in tensile strength then proceeded from 5% to 7% BA concentration which is 49.98 MPa to 47.13 MPa. The control samples with 0% BA concentration have a mean tensile strength value of 41.98 MPa which is the lowest compared to all samples impregnated with BA.
The highest mean compression strength parallel to fiber grains, Rc in test 3 is 59.78 MPa with 3% BA concentration and the lowest mean value is 55.34 MPa with 5% BA concentration. The mean compression strength shows an increase from 3% to 5% BA concentration which is 59.78 MPa to 55.34 MPa. An increase in compression is shown from 5% to 7% BA concentration which is 55.34 MPa to 59.22 MPa. The control samples with 0% BA concentration have a mean compression strength value of 64.14 MPa which is the highest amongst all the impregnated samples.
Comprehensive studies on the mechanical properties of waterborne preservatives have been conducted in the 1980s and 1990s which are used as reference studies for research today. Of which, Winandy (1995) has contributed greatly to this with his compressive study on the effects of waterborne preservative treatment on mechanical properties which contains key research data on different effects of wood treated with waterborne preservatives. For that study, it states that bending strength, MOR typically reduces from 0 to 20 percent after treated with waterborne preservative. This holds true as for 3% and 5% BA concentration, the mean MOR values reduced approximately by 16%. The magnitude of this decrease can be attributed to the preservative chemistry and redrying temperature of the treatment. In a separate study conducted by Winandy et al. (1992), CCB treated wood kiln-dried at 71°C and 116°C showed a general decrease in MOR and concluded that wood initially dried at high-temperature and redried at any subsequent temperature will result in strength loss. The drying and redrying conditions for the current study are equal at 103 ± 2°C which partially fits the description as the MOE values of impregnated samples are greater than that of the control samples. On the other hand, the effects on MOE or stiffness are reversed that of MOR. High-temperature initial drying and subsequent redrying increases the MOE values and this phenomenon of increased stiffness and decreased strength (MOR) is called embrittlement of material. It can be concluded that this drying combination exaggerates the effect of waterborne preservative treatment.
Other research shows that waterborne preservatives have a negative impact on mechanical properties of treated wood as the chemicals physically react with the wood cell wall material (Furuno and Goto, 1978). Preservatives such as BA containing acidic chromium induces a hydrolytic depletion or fixation reaction in the wood sugars causing metals to reduce to a less water-soluble state by oxidation of the components of cell wall. This leads to inconsistent results in mechanical properties (Temiz et al., 2004). Research by Toker (2007) that involved treating Calabrian pine and Oriental beech wood with various concentrations of aqueous borate solution concluded that there was a statistical difference in compression strength parallel to grain values between control and treated samples. A report by Simsek et al. (2010), shows that borate treatments decrease the compression strength parallel to the grain by 3%–41% and 7%–22% for Oriental beech and Scots pine, respectively. Oriental beech and Scots pine are hardwoods and softwoods respectively which provides good reference points to compare the preservative to compression strength parallel to the grain relationship with LRM.
Mechanical properties of small pieces of timber known as clear specimens such as MOE, MOR, tensile and compression strength tend to be higher than their structurally larger counterpart. As shown in a study conducted by Azlan et al. (2018) where most stress results provided by the structural specimen, Kapur, Merpauh, Resak, Bintangor, White Meranti, Jelutong, and Kelempayan were lower than the small clear specimen expect for Sesendok. Hence, application of standard grade stress reduction ratios provided such as from Chu et al. (2013) may be inaccurate due to differences in structural sizes of timber. Another study by Winandy (1995) suggests that materials of larger size particularly larger thickness will have a smaller strength reduction compared to smaller-sized materials. It was said that this effect is a function of the surface-area-to-volume ratio of each product. For instance, at similar preservative retention levels, poles and piles typically have less strength reduced compared to lumber.
From Table 6, the statistical analysis of the data using the ANOVA test indicates that there are significant interaction effects at 5% level of significance in MOE between BA concentration and different samples of the same size as indicated by the p-value of 0.000627. This is not the case for MOR, Rt, and Rc as the p-values and F-values are greater than 0.05 and less than the F-crit values respectively. This shows that there is no statistical significance. The lack of statistical significance of three out of the four results can be attributed to two main factors namely sampling bias and species-specific differences. The samples were all obtained from a local woodworking mill in Miri from a specific production period which indicates that the data set was not diverse. The origin of the meranti species obtained was naturally grown and its quality may differ from those that are plantation-grown (de Souza Almeida et al., 2019). Additionally, the presence of pith has been proven to have substantial effect on strength. It is worth noting that mechanical properties are affected differently depending on the condition of treatment. For instance, the mechanical property reduction for wood is typically insignificant for preservative retention levels of less than 16 kg/m3 and redrying temperatures less than 71°C (Winandy et al., 1992).
The ANOVA test revealed the statistical insignificance of the increase in Rt and reductions in MOR and Rc values compared to their respective control samples. The statistically insignificance of the increase in Rt is consistent with the findings of Winandy et al. (1992) as they concluded that a consistent but statistically insignificant, tensile strength reduction of 7% to 10% above the 25th percentile. The only difference is the increase in tensile strength with increasing preservative concentration of this study while the opposite is true for the study conducted by Winandy et al. (1992).
This can be attributed to the differences in treatment condition namely dimension, type, and treatment of the wood.
The other study presented a more holistic analysis on the effects of different combinations of pre- and post-drying temperatures, duration of treatment, and drying schedules. However, in this study, the limitation on the variety of treatment conditions questions the pertinence of this data to previous data.
Potential discrepancies between theoretical and experimental values may appear due to the difference in loading nature.
For instance, in a three-point bending test, force is concentrated on a singular center point on the wood which does not reflect forces applied on wood in real life applications. In addition, wood as an anisotropic material performs better under uniaxial stress. Most of the destructive tests highlighted in this research are uniaxial as forces only act in a uniaxial plane. This also is a cause for discrepancy as wood is typically subjected to forces from various directions especially in real life applications (University of Cambridge, 2023). It should be noted that the magnitude of effect of various water-borne preservatives on mechanical properties does not deviate greatly between different species (Cha et al., 2022). Hence, references can be taken from preceding case studies of similar wood type (Green et al., 1999).
The highest mean compression strength parallel to fiber grains, Rc in test 3 is 59.78 MPa with 3% BA concentration and the lowest mean value is 55.34 MPa with 5% BA concentration. The mean compression strength shows an increase from 3% to 5% BA concentration which is 59.78 MPa to 55.34 MPa. An increase in compression is shown from 5% to 7% BA concentration which is 55.34 MPa to 59.22 MPa. The control samples with 0% BA concentration have a mean compression strength value of 64.14 MPa which is the highest amongst all the impregnated samples.
Research shows that waterborne preservatives have a negative impact on mechanical properties of treated wood as the chemicals physically react with the wood cell wall material (Furuno and Goto, 1978). Research by Toker (2007) that involved treating Calabrian pine and Oriental beech wood with various concentrations of aqueous borate solution concluded that there was a statistical difference in compression strength parallel to grain values between control and treated samples. A report by Simsek et al. (2010), shows that borate treatments decrease the compression strength parallel to the grain by 3%–41% and 7%–22% for Oriental beech and Scots pine, respectively. Oriental beech and Scots pine are hardwoods and softwoods respectively which provides good reference points to compare the preservative to compression strength parallel to the grain relationship with LRM.
Mechanical properties of small pieces of timber known as clear specimens such as MOE, MOR, tensile and compression strength tend to be higher than their structurally larger counterpart. As shown in a study conducted by Azlan et al. (2018) where most stress results provided by the structural specimen, Kapur, Merpauh, Resak, Bintangor, White Meranti, Jelutong, and Kelempayan were lower than the small clear specimen expect for Sesendok. Hence, application of standard grade stress reduction ratios provided such as from Chu et al. (2013) may be inaccurate due to differences in structural sizes of timber.
From Table 6, the statistical analysis of the data using the ANOVA test indicates that there are significant interaction effects at 5% level of significance in MOE between BA concentration and different samples of the same size as indicated by the p-value of 0.000627. This is not the case for MOR, Rt, and Rc as the p-values and F-values are greater than 0.05 and less than the F-crit values respectively. This shows that there is no statistical significance.
Meranti is typically used for indoor and outdoor applications due to its workability and machinability, and moderate durability. Treating meranti with BA has varying effects on both the physical and mechanical properties. The current study presents mean retention rates of above 8 kg/m3 which is the standard to effectively protect against insectile and fungicidal infestation excluding lower BA concentrations (3% BA of test 1 and test 3). This indicates that LRM has sufficient retention rate at higher BA concentrations to protect against attacks from insects and fungi especially since LRM is highly susceptible to lyctic borers and termites (Wood Solutions, 2024). For reference, a weight loss of 5%–10% will result in a decrease of mechanical properties from 20% to 80% (Kim et al., 1996). The retention rate of wood is a function of SAV ratio as higher SAV will result in higher retention rates and vice versa. Hence, this relationship should be taken into consideration when devising a treatment plan for timber products against biological deterioration (Priadi et al., 2021).
The structural use of timber is typically classified according to their strength and stiffness (MOE). The enhanced mechanical properties in terms of the MOE, the greater stiffness after treatment will increase the viability of using LRM for more construction and structural applications (Arriaga et al., 2023). In the current study, the Rc or compression strength parallel to the grain is measured in the longitudinal direction which is the typical orientation of wood during application. However, for most real-life applications, forces may be applied in various directions and magnitudes depending on the situation. The general decrease in Rc of BA treatment indicates that this treatment may not be suitable especially for applications where having high compression strength is necessary. The same can be said for the other mechanical properties when being applied in real life situations. However, the opposite is true for MOE and Rt as they showed a general increase in value which suggests their viability in fields where these values are important.
Under similar conditions, the results obtained using one type of waterborne preservative may be used as a reference point for waterborne preservatives in general as the magnitude of effect of different waterborne preservatives differ slightly compared to other factors such as treatment processing factors (Priadi et al., 2020). The differences that appear can be attributed to the reactivity of preservative and temperature of treatment. The same is not true for scaling the dimensions of wood. Larger samples tend to have proportionally reduced values of mechanical properties compared to their smaller counterparts. However, when measuring overall reduction in mechanical properties, smaller samples are subjected to greater reductions compared to larger samples (Green et al., 1999).
4. CONCLUSIONS
This study presents the influence of BA on the physical and mechanical properties of LRM impregnated using the dip diffusion method. The physical properties, particularly retention rate have been shown to increase because of the boron treatment. The main contribution from this study is that the boron treatment manages to improve the mechanical strength in terms of the MOE and Rt parameters. However, the boron treatment has been shown to decrease the mechanical strength in terms of the MOR and Rc parameters. The ANOVA test indicates for retention rate and MOE, there are significant interaction effects between all the factors tested. On the other hand, the ANOVA test also indicates that there is no statistical significance for the values measured for MOR, Rt, and Rc as indicated by the p-value being greater than a 5% level of significance.
Further recommendations for this research project include experimenting with different boron compounds with a larger sample size on the mechanical properties and impregnation methods. BA is one of many waterborne preservatives used to treat timber-products, these include, borax, CCB, tannin, CCA, and ammoniacal-based (ACZA and ACQ). Different compounds are known to vary in their effects on physical and mechanical properties of timber-products, especially meranti. Studies by J. E. Winandy, shows that waterborne preservatives tend to decrease mechanical properties of timber-products. However, studies have shown that this is not always the case as properties such as MOE and crushing strength are generally unaffected (Winandy, 1995).
Impregnation methods also play a vital role in ensuring that the depth of penetration is sufficient for effective treatment. Besides the dip diffusion method used in this report, the pressurized method, full-cell treatment is a popular choice used in industries to treat timber-products such as planks. It provides consistent and sufficient preservative penetration for various wood species that are not difficult to treat. Additionally, further testing on the absorption of preservative into LRM can be performed as LRM is a hardwood and absorption at higher preservative concentration is difficult. A case study can be made to compare the influence of different boron compounds with various concentrations along with a different preservative treatment method for difficult to treat wood such as meranti. Further increasing understanding on the influence that boron compounds have on wood such as meranti.