1. INTRODUCTION
Indonesia is a tropical country and is known as the third largest mega-biodiversity country in the world (Savero et al., 2020). At least, 4,000 tree species exist in the country’s tropical rainforests (Martawijaya et al., 2005). One of wood species that is quite popular in Indonesia is mahogany (Swietenia mahagoni). In Indonesia, it is a popular raw material for veneer decorations, plywood, and furniture (Martawijaya et al., 2005). The heartwood of S. mahagoni is resistant to decay and insect attack and performs better on the global market than Swietenia macrophylla and Swietenia humilis (Sarhean et al., 2015). Febrianto et al. (2015) stated that mahogany wood was included in durability class II. Arinana et al. (2022) reported that the wood of S. mahagoni was the last of the six species of wood preferred by the subterranean termites. Cahyono et al. (2020) also found that laminates made from S. mahagoni wood veneers were the most resistant to drywood termite attack. Furthermore, Yanti et al. (2019) obtained that the modulus of elasticity and modulus of rupture values of oriented strand board increased after being coated with wood veneer from S. mahagoni. Tannin resorcinol formaldehyde prepared from mahogany bark extract was also reported to be more environmentally friendly due to low formaldehyde emissions (Trisatya et al., 2023). The mahogany heartwood is the most marketable component for which international timber markets expect premium payments (Wadsworth and González, 2008). The presence of heartwood is an important factor in determining the grade and its potential use (Seta et al., 2023). The colour of mahogany heartwood is reddish or pink, and darkens with age to dark red or brown. Genetic factors are thought to be the main reason for the emergence of variation in heartwood colour. However, not all heartwood with similar genes will show the same colour (Won et al., 2017). Genetic factors are also thought to control growth characteristics (tree height, diameter and volume) and basic density (Fadwati et al., 2023).
According to the International Association of Wood Anatomists (IAWA, 1964), heartwood is wood that no longer contains living cells in growing trees where reserve materials such as starch have been converted into heartwood substances. Meanwhile, sapwood is wood that contains living cells in living trees and has reserve materials such as starch (Anonymous, 1957). Parenchyma cells in the transition zone between sapwood and heartwood undergo metabolic changes prior to cell death (Piqueras et al., 2020). This results in increased synthesis of secondary metabolites called extractives. Extractives, a naturally occurring minor component of wood, are essential in protecting wood from decay by wood-destroying organisms (Darmawan et al., 2018). Previous studies have also reported the potential biological activity of extracts soluble in various polar solvents (i.e. methanol, ethanol, and distilled water) from several species such as Azadirachta excelsa seeds and Guibourtia tessmanii bark for anti-termite (Adfa et al., 2023; Ella Nkogo et al., 2022), herbal plants of Phellodendri cortex, Magnoliae cortex and some plants for antifungi (Na and Kim, 2022; Yoon and Kim, 2023), 12 herbal plants for antimicrobial resistance (Ham and Kim, 2022), Quercus gilva and Citrus × natsudaidai for antioxidant activity (Huh et al., 2022; Yang et al., 2022a) and the Lauraceae family for anti-asthmatic activity (Yang et al., 2022b).
Several basic properties play an important role in the utilization of wood as a raw material, one of them is information about the characteristics of the heartwood (Iswanto et al., 2021). The process of heartwood formation is known to be associated with storage depletion, parenchyma cell death, and increased extractives (Hillis, 1987). The depletion of reserves, starch, non-structural carbohydrates (NSC) and triacylglycerol is a form of parenchyma cell disintegration. In the outer sapwood, which contains many starch granules, starch gradually decreases to the transition zone. Starch in the middle sapwood is hydrolysed by phosphatases to sugar phosphates and thus becomes a precursor for the synthesis of extractives or lipids. Small lipophilic droplets were found in the axial and ray parenchyma of the outer sapwood. These values were significantly increased in the middle and inner sapwood as well as in the transition zone. Lipids and carbohydrates in these tissues are thought to provide the carbon skeleton for extractive synthesis in heartwood (Kampe and Magel, 2013). Heartwood is known to be free of storage material. Therefore, during heartwood formation, starch and lipid are degraded and play an important role as carbon skeletons for extractive phenolic biosynthesis and senescence of ray parenchyma cells (Hillis, 1987; Magel, 2000; Song et al., 2011).
It is well known that lipids such as fatty acids, resin acids and sterols have been analysed to find out their involvement in the process of heartwood formation. In some previous studies, it was known that the amount of fatty acids and sterol composition increased from sapwood to heartwood due to the heartwood formation process in some species such as Pinus radiata and Pinus sylvestris (Hemingway and Hillis, 1971; Hillis, 1972; Höll and Goller, 1982; Kampe and Magel, 2013; Saranpää and Nyberg, 1987). In addition, the extractive composition of heartwood was greater than that of sapwood (Hillis, 1971; Miranda et al., 2006). The accumulation of extractives in the heartwood is a key feature of heartwood formation (Hillis, 1971).
Most sterols in plants are found in cellular organelles (Grunwald, 1970) and in the plasmalemma (Hartmann et al., 1975). It has been suggested that the interaction of sterols with phospholipids stabilises the membrane. This will be control permeability (Grunwald, 1980). Sterol levels were found to be higher in heartwood than in sapwood (Höll and Goller, 1982). To the best of our knowledge, there is no study of the lipophilic extractives in S. mahagoni wood associated with heartwood formation. Therefore, the aim of this study was to identify the lipophilic extractives and their proportion in relation to heartwood formation in S. mahagoni.
2. MATERIALS and METHODS
For the preliminary studies, the 4-year-old trees had been collected in December 2018, while the 2- and 3-year-old trees had been obtained in October 2019. In the meantime, the 1- and 5-year-old trees were felled in October 2020. Three different trees from the lower part (5% of the total merchantable height) were sampled for each tree age. Young tree samples (1–5 years old) were harvested from the Perum Perhutani plantation in Temanggung Regency, Central Java, Indonesia (7°14′S, 110°2′E) during the rainy season. Table 1 describes the tree age, diameter at breast height and total merchantable height.
At one to three years of age, visual inspection of the mahogany trunk in cross section showed no signs of discolouration. Meanwhile, at the age of four years a colour change occurred with a distinct salmon pink to red colour in comparison to the light-coloured sapwood. The area of heartwood formation was then identified at this colour change. Samples from the 1- to 3-year-old trees were taken from the sapwood only as they heartwood areas were not present. Subsequently, the disc samples were stored at room temperature and air-dried. Disc samples in the cardinal direction were then drilled to sample the wood. Samples of sapwood (0.5 cm from the bark-sapwood boundary) and heartwood (1.0 cm from the sapwood-heartwood boundary) were collected for chemical analysis. The drilled wood was then separately ground into powder and sieved (through a 1 mm sieve) for chemical analysis.
Powders of 0.8 g (1-year-old plants) and 1.5 g (2- to 5-year-old plants) were extracted with n-hexane (60°C for 6 h) in a Soxhlet apparatus. There were 8 cycles per hour during the extraction process. The solvent was dried by evaporation and the weight of the extract is expressed as a percentage of dry wood.
The lipophilic constituent was identified by Gas Chromatography-Mass Spectrometry (GC-MS-QP 2010, Shimadzu, Kyoto, Japan) under the following conditions: RTX-5MS capillary column (30 m × 0.25 mm I.D. and 0.25 μm; GL Sciences, Tokyo, Japan); carrier gas He; injector temperature 200°C; oven initial temperature from 70°C (2 min) to 290°C at 5°C/min; detection temperature 285°C; acquisition mass ranged from 50 to 666 amu. The method used trimethylchlorosilane (TMCS) derivatization and the samples were derivatized before injection according to the method described by Moldeveanu and David (2018) with modifications. Furthermore, a mixture of 100 μL N,O-bis-(trimethylsilyl)-trifluoroacetamide (BSTFA) and 1% TMCS was added to 2 mg of each dry n-hexane extract diluted in 100 μL pyridine. Before injecting 1 μL (1 mg/mL) into the GC-MS, 1 mL of n-hexane solution was added to the mixture and sonicated at room temperature. The compounds were identified by comparing experimental GC-MS data with authentic compounds, the NIST MS library (NIST, 2011), and research literatures (Arisandi et al., 2020; del Río et al., 2011; Domingues et al., 2010; Ferreira et al., 2018; Freire et al., 2002a, 2002b, 2006a, 2006b; Gutiérrez et al., 1999, 2006; Hafizoğlu et al., 2002; Silvério et al., 2007).
β-sitosterol was used to prepare a calibration curve (Fig. 1) for the quantification of sterol fractions with a concentration range of 0.2, 0.1, 0.05, and 0.00625 μg/μL (R2 = 0.995). For the quantification of fatty acids, palmitic acid was used to obtain the calibration curves (Fig. 2) at the range of 0.3, 0.1, 0.05, 0.01, 0.0025, and 0.000625 μg/μL (R2 = 0.999). The respective response factors were calculated as the average of three GC–MS runs, and other constituents were compared to internal standards, such as veratric acid.
Standard compounds, including, dodecanoic, myristic, pentadecanoic, palmitic, oleic, linoleic, stearic, arachidic, docosanoic, and tetracosanoic acid were obtained from Shanghai Chemical Industry (Shanghai, China). Meanwhile, veratric acid and β-sitosterol were purchased from Kanto Chemical (Tokyo, Japan).
3. RESULTS and DISCUSSION
The total extractive content of n-hexane extract was from 0.76% to 2.45% based on dried wood (Table 2). Non-polar solvents such as n-hexane, dichloromethane, and chloroform, are commonly used for the analysis of lipophilic compounds (Arisandi et al., 2020; Freire et al., 2006a, 2006b; Gominho et al., 2020; Gutiérrez et al., 1999; Silvério et al., 2007). A total of four lipophilic groups were detected in the species examined, namely hydrocarbons, fatty acids, sterols, and others (Table 2, Fig. 3).
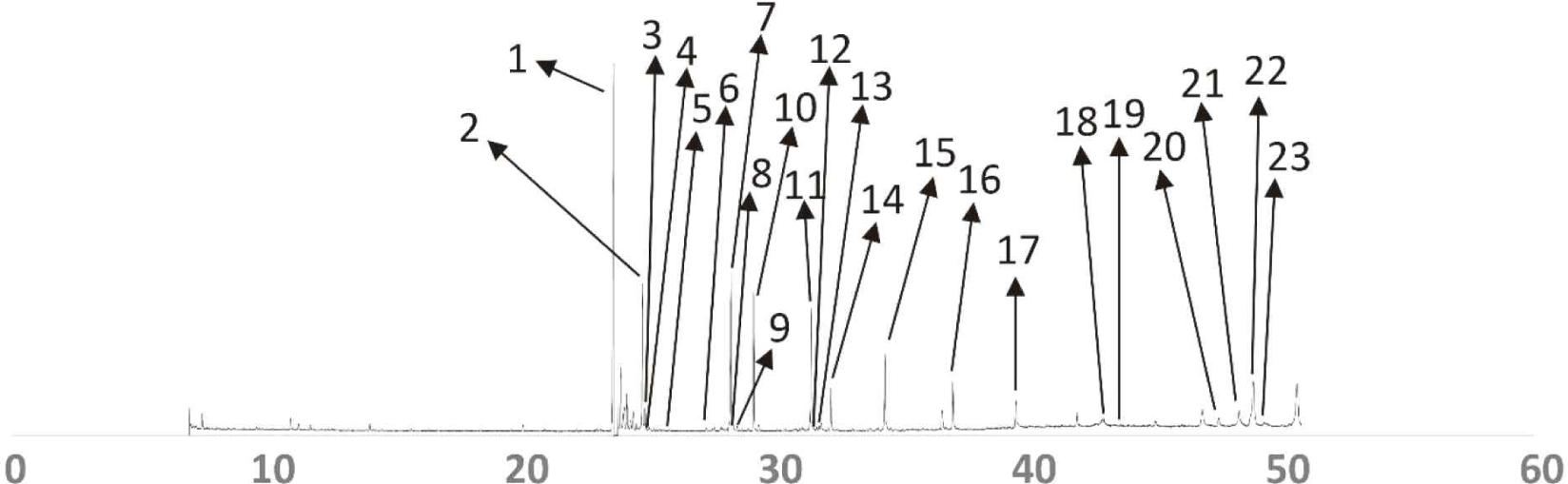
The abundant hydrocarbon compounds were pentadecane, 1-octadecene, hexadecane, cyclotetracosane, cycloeicosane, and cyclooctacosane. Meanwhile, the few compounds were cetene, undecane, 3-methylene-, tridecane, 3-methylene-, heptadecane, and heneicosane. In other species such as S. macrophylla in the bark part, Arisandi et al. (2019) reported that 1-octadecane, pentadecane, and cyclooctacosane were the most abundant, while heptadecane was detected as a minor component. In addition, 1-tridecane and 1-octadecane were recorded in Eucalyptus globulus (Gominho et al., 2020). The hydrocarbon fraction in this study was indeed the largest. It belongs to the lipophilic group with a large amount in the wood, as reported by some studies in E. globulus species (Gutiérrez et al., 1999; Swan, 1967).
Fatty acid groups, namely, dodecanoic, myristic, pentadecanoic, palmitic, oleic, linoleic, stearic, arachidic, docosanoic, and tetracosanoic acids were detected in the S. mahogani wood. Other studies such as in E. globulus, Eucalyptus urograndis, Eucalyptus pellita, Eucalyptus dunnii, and Eucalyptus grandis × Eucalyptus urophylla clone wood, also reported the same compounds (Arisandi et al., 2020; Silvério et al., 2007). In S. mahagoni, palmitic, linoleic, oleic, and stearic acid compounds were the main fatty acid groups. Some studies also reported the same composition in other species, such as E. globulus, E. pellita, E. grandis, E. dunnii and E. grandis × E. urophylla clones (Arisandi et al., 2020; Freire et al., 2002b; Gominho et al., 2020).
The sterol group was the second largest fraction after the formation of heartwood (Table 2, Fig. 4). In previous studies, sterols were mostly the second largest fraction as reported in E. pellita, E. grandis, E. dunnii, and E. grandis × E. urophylla clone (Arisandi et al., 2020; Kilulya et al., 2014). In S. mahagoni, the abundant sterol composition includes stigmasterol, β- sitosterol, campesterol, and cyclolaudenol. Meanwhile, components of urs-12-en-28-ol, stigmast-5-en-3-ol, oleate cycloeucalenol were the minor compounds. From these constituents, β-sitosterol was the largest compound in the sterol fraction. Similar results were also reported in previous studies such as on E. globulus clone, E. pellita, E. grandis × E. urophylla clone (Arisandi et al., 2020; Freire et al., 2002a, 2002b; Gominho et al., 2020). According to Rastogi and Mehrota (1993), mahogany heartwood contains β-sitosterol, lupleol, cyclo swietenol, benzoic hedergenin, and cycloartenol.
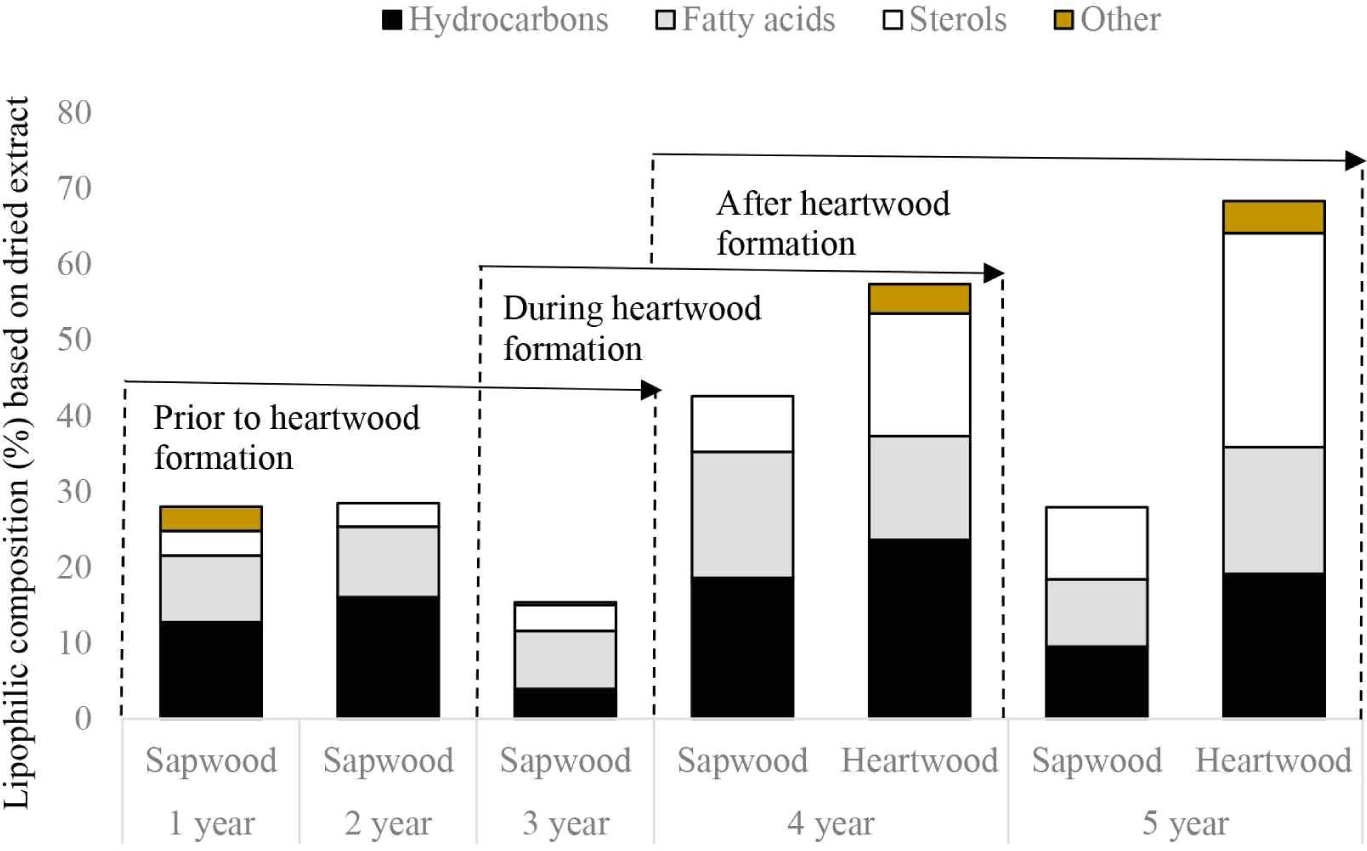
Before heartwood formation (3 years), lipid groups of hydrocarbons increased from 1- to 2-year-old and then decreased drastically to 3-year-old namely 1-octadecene, cyclotetracosane, cycloeicosane, and pentadecane (Table 2, Fig. 4). Meanwhile, the amount of hexadecane, heneicosane, and cyclooctacosane decreased from 1- to 3 years old. Furthermore, the number of fatty acids increased from 1- to 2-year-old and decreased to 3-year-old, specifically for palmitic and linoleic acids. The amount of oleic acid increased from 1 to 3 years old, and a reverse trend was found in the content of stearic acid. Dodecanoic, myristic, and pentadecanoic acid were only detected at the age of 1 year old. In addition, sterols content slightly increased from 1 to 3 years, even though the amount of β-sitosterol decreased. A similar trend was also shown in another compound known as 1-heneicosanol.
During heartwood formation (3 to 4 years), hydrocarbon components increased four times in the sapwood part. Components that contributed greatly to the increase in this number were pentadecane, and 1-octadecane. In addition, fatty acids and sterols increased twice. The fatty acid components that contribute to increasing levels are linoleic, oleic, and stearic acids. Sterol components are β-sitosterol, campasterol, and cyclolaudenol. The opposite pattern was found in the 1-heneicosanol.
After heartwood formation at 4-year-old, the level of hydrocarbon and fatty acid contents decreased almost twice times in the sapwood (Table 2, Fig. 4). This was because the components of pentadecane, hexadecane, heptadecane, and heneicosane in the sapwood decreased dramatically at the age of 5 years for hydrocarbons fractions, while the fatty acid groups contained palmitic, linoleic, and oleic acid. In the heartwood, generally all fractions increased (except for the hydrocarbon fraction). The composition of sterol fraction almost doubled from 16.2% to 28.2%. After the formation of heartwood, the largest fraction was hydrocarbons, followed by sterols and fatty acids. These results are similar to a study conducted by Silvério et al. (2007) and Gutiérrez et al. (1999) on E. urograndis clones and E. globulus respectively. The results showed that hydrocarbons, fatty acids, and sterols were the main lipid groups identified.
Decreasing or increasing in some extractive compounds due to the age of the tree is influenced by several factors. The extractive content increases, it is thought to be because the cambium vessels in older trees are more likely to divide and multiply into parenchyma cells, so that the formation of parenchyma cells increases. Therefore, the extractive content tends to increase (Hamidah et al., 2009). The similar pattern had been reported in previous studies in E. globulus, E. grandis, E. urophylla, and Tectona grandis (Lestari et al., 2016; Lukmandaru, 2009; Miranda and Pereira, 2002; Santana et al., 2012). The levels of extracts or compounds drop, this may be because the compounds are converted to other secondary compounds for heartwood formation or other physiological processes, such as respiration, photosynthesis, etc.
The fatty acid composition of the heartwood differed from that of the sapwood (Table 2, Fig. 4). The sapwood was rich in fatty acids with 16.70%, while the heartwood part was 13.70% at 4 years of the tree. Even though, the compound of palmitic acid represented 6.08% in the sapwood, while in the heartwood it was only 6.27%. Fatty acid composition tended to decrease from sapwood to heartwood, including unsaturated fatty acids (oleic and linoleic). Saranpää and Nyberg (1987) reported that the amount of oleic (18:1) and linoleic (18:2) acids in the triacylglycerol fraction decreased towards the heartwood. This suggests a general unsaturation of the triacylglycerols during heartwood formation. A similar pattern was also reported by Bertaud and Holmbom (2004) and Ekman (1979), where the amount of lipophilic extractives was greater in sapwood than in heartwood of Picea abies. This may be due to the greater number of triglycerides. Triglycerides and sterile esters are present in living parenchyma cells. As heartwood forms and parenchyma cells die, triglycerides are hydrolyzed by lipase enzymes. However, triglycerides were not quantified in this study and further research is needed in this case. Hemingway and Hillis (1971) also found that the metabolized glyceride esters were rich in oleic acid (18:1), whereas linoleic acid (18:2) and saturated fatty acids were concentrated in other esters, such as wax in P. radiata. Another possibility is that at 4 years, heartwood was just beginning to form, so that the lipids in the sapwood have not all been fully metabolized into heartwood extractives such as fatty acids. Magel et al. (1991, 1994) reported analyses of heartwood extractives on individual tree rings from the cambium to the pith. They found that the physiological process of heartwood formation starts on the third or fourth growth and was completed after 2 years. Dünisch et al. (2010) also stated that the extractive content of heartwood was higher in the mature heartwood (9.80%–10.30%) than in the heartwood formed during the juvenile phase. Hillinger et al. (1996) and Magel et al. (1991) mentioned that carbohydrates and lipids were soluble in methanol in the sapwood part of black locust. The living tree uses these substances especially during the process of heartwood formation.
There were marked differences in the fatty acid composition of sapwood and heartwood between 4- and 5-year-old trees (Table 2, Fig. 4). At 5 years of age, almost all fatty acid compounds, including unsaturated fatty acids (oleic and linoleic) increased from sapwood to heartwood. Heartwood contained 16.80% of fatty acid content while sapwood contained 8.89%. Similar trends have been reported by some previous studies in several other species such as E. globulus (Gominho et al., 2020), Eucalyptus camaldulensis (Benouadah et al., 2017), Acacia hybrid (Soon and Chiang, 2012), Pinus sylvestris (Saranpää and Nyberg, 1987) and P. radiata (Hemingway and Hillis, 1971). It is clear, therefore, that by the age of 5 years, most of the lipids have been converted to extractive compounds in the heartwood. Since ray parenchyma cells have starch-containing plastids (amyloplasts; Parameswaran and Bauch, 1975), it is reasonable to assume that the fatty acids and lipids found in wood are at least partly synthesized in living wood cells and not transported from the photosynthetic cells of the tree. Plastids are thought to be the sole source of fatty acids in plants (Stumpf, 1980). However, the fatty acids present in wood do not always appear to be identical to those present in leaves, and their relative amounts may be very different.
Other fatty acids such as dodecanoic, myristic and arachidic acids were only found in sapwood. In contrast, long chain fatty acids such as docosanoic and tetracosanoic acids were only found in the heartwood. Arisandi et al. (2020) also reported that long chain fatty acids such as docosanoic and tetracosanoic acids were more abundant in the heartwood than in the sapwood of E. pellita species. The concentration of fatty acids in heartwood samples, especially long-chain fatty acids suggests that these compounds are produced extensively during heartwood formation as a defence mechanism against microorganisms and insects or fungal decay (Blanchette and Biggs, 1992; Metsä-Kortelainen and Viitanen, 2009). On the other hand, fatty acids appear to have antibacterial activity (Desbois and Smith, 2010). Fatty acids can act as ionic surfactants and have antibacterial and antifungal properties at low pH (Hayes and Berkovitz, 1979). Palmitic, stearic, oleic, linoleic and linolenic acids are well known to have antibacterial antioxidant activities (Karimi et al., 2015; McGaw et al., 2002).
The composition of hydrocarbons in sapwood differed from that in heartwood. In general, the content of hydrocarbons (including major compounds) was higher in heartwood than in sapwood at both 4 and 5 years of age. Hydrocarbon composition in the sapwood was 18.60% and 9.51%, whereas in the heartwood it was 23.60% and 19.10% at 4 and 5 years of age, respectively (Table 2). Hydrocarbons like fatty acids and sterols are lipophilic compounds. Hydrocarbons are commonly found in wood, for example in eucalyptus wood. del Río et al. (1998) reported that in E. globulus wood extractives consist mainly of hydrocarbons, fatty acids, waxes, steroids and triglycerides. There is very little discussion on the hydrocarbon composition of sapwood and heartwood (Gominho et al., 2020), except for the general hydrocarbon composition of wood, bark and pulp (del Río et al., 1998; Gominho et al., 2020; Gutiérrez et al., 1999, 2006; Kilulya et al., 2012, 2014). The function of hydrocarbons in relation to the heartwood formation process may be similar to that of fatty acids and sterols as they are still in the same lipid class. Previous research indicated that four hydrocarbon compounds, including octacosane, tetracontante, hentriacontane and heneicosane, are act as antifungal agents on Cryptococcus neoformans and Phanerochaete chrysosporium (Masendra et al., 2015; Passos et al., 2003). However, the more specific role of hydrocarbons in the process of heartwood formation needs to be further investigated.
The highest number of sterols was found in the heartwood (Table 2, Fig. 4). The composition of the sterol fraction in sapwood at 4 and 5 years of age was 7.36% and 9.51%, respectively. Meanwhile, in heartwood was 16.20% and 28.20%, respectively. It is interesting to note that the heartwood, which was mostly dead, contained the highest concentration of sterols (Fig. 4). The main sterols detected were stigmasterol, β-sitosterol, campesterol and cyclolaudenol of which β-sitosterol was the largest compound in the sterol fraction with a composition of 3.27% to 9.04%. In addition, compounds such as urs-12-en-28-ol and stigmast-5-en-3-ol, oleate, cycloeucalenol (4 years) were only detected in the heartwood. The increase in sterol compounds from the sapwood to the heartwood region is similar to that reported in previous studies in several species, such as E. globulus (Gominho et al., 2020), E. camaldulensis and Pinus halepensis (Benouadah et al., 2017), and Acacia hybrid (Soon and Chiang, 2012). This increase in free sterols in heartwood may be due to their involvement in self-defence mechanisms (Hillinger et al., 1996). Höll and Goller (1982) also found the highest concentrations of free sterols and sterol esters in the inner heartwood of spruce and suggested that these compounds are synthesized when the tree is young. Höll and Lipp (1987) suggested that sterols accumulate in the heartwood of Pinus sylvestris during the early stages of the life cycle. Another possibility is the amount of sterol ester compounds hydrolyzed during the heartwood formation, as occurs in P. abies (Bertaud and Holmbom, 2004).
It can be said that different developmental stages of parenchyma cells have certain characteristics in their lipid metabolism. In observations on the species Robinia pseudoacacia, Kampe and Magel (2013) explained that the amount of phospholipids and parenchyma cell integrity parameters decreased towards the transition zone. Only trace amounts were found in the heartwood. The amount of free sterols decreased from sapwood to the transition zone, but reached a maximum in the heartwood. According to the researchers, the first stage of cell damage may be due to a decrease in both compounds. The increase in free sterols in heartwood may also be due to a possible involvement in defence mechanisms against pathogens (Hillinger et al., 1996). For example, the compound of β-sitosterol is known to have antifungal activity against Fusarium spp. (Kiprono et al., 2000) and inhibits the growth rate of the fungus P. chrysosporium (Masendra et al., 2020). Steroid constituents such as cycloeucalenyl acetate and stigmasta-3,5- diene from n-hexane extract of E. pellita have been specifically associated with increased toxicity to Cryptotermes termites (Masendra et al., 2021). A high accumulation of steroid compounds was also believed to affect potent antioxidant activity (Masendra et al., 2021). Furthermore, phytosteroids may be useful in treating inflammatory diseases, such as inflammatory bowel disease, rheumatoid arthritis, multiple sclerosis, asthma, and cardiovascular disease (Cheng et al., 2022).
The depletion of reserve substances, starch, NSC and triacylglycerol has previously been recognized as a marker of parenchyma cell disintegration (Kampe and Magel, 2013). The abundant starch granules in the outer sapwood gradually decrease from the middle sapwood towards the transition zone. The starch contained in the middle sapwood is hydrolyzed by phosphatases into sugar phosphates, thus becoming a precursor for lipid or extractive synthesis. The researchers also found lipophilic spots in the axial parenchyma and spokes in the outer sapwood. These spots increased significantly in size in the middle and inner sapwood cells and in the transition zone. Within these tissues, lipids and carbohydrates are thought to provide the carbon skeleton for the synthesis of heartwood extractives. Thus, lipids and starch are degraded during heartwood formation and play an important role in providing the carbon skeleton for the biosynthesis of phenolic extractives and thus the ageing of ray parenchyma cells (Hillis, 1987; Lamlom and Savidge, 2006; Magel, 2000, Song et al., 2011). This is consistent as reported by Arisandi et al. (2022), where the main soluble sugar compounds, monosaccharides (fructose, glucose, mannose and xylose) and disaccharides (sucrose) decreased from sapwood to heartwood. Even the compounds of arabinose, galactose and sucrose at 5 years old were found only in sapwood in S. mahagoni.
4. CONCLUSIONS
In conclusion, the present study was the first report on the lipophilic components found in the sapwood and heartwood of S. mahagoni, specifically regarding their relevance to heartwood formation. The n-hexane extract content varied between 0.76% and 2.45%, based on the weight of dried wood. Furthermore, four lipophilic fractions including hydrocarbons, fatty acids, sterols, and other compound (1-heneicosanol) were identified in S. mahagoni wood. The major groups of constituents consisted of sterols (β-sitosterol, stigmasterol, campasterol, and cyclolaudenol), fatty acids (palmitic, oleic, linoleic, and stearic acids), and hydrocarbons (pentadecane, 1-octadecane, hexadecane, cyclotetracosane, cycloeicosane, and cyclooctacosane), while 1-heneicosanol was minor compound. After heartwood formation, the hydrocarbon fraction was the largest followed by sterols, fatty acids, and 1-heneicosanol. Lipophilic extractives increased with tree age, except for the hydrocarbon fraction which decreased at 3 and 5 years (sapwood). Furthermore, the distribution of lipophilic extractives increased drastically from sapwood to heartwood, especially at 5 years, except for fatty acids at 4 years, which showed the opposite trend. It is suggested that at the age of 4 years, lipids were not fully converted to heartwood extractives at 4 years of age, when heartwood was just being formed. In addition, the role of hydrocarbons in relation to heartwood formation needs to be further investigated.