1. INTRODUCTION
Wood is utilized for numerous applications, including the construction of houses, furniture, and household furnishings. Wood is typically chosen as a primary material due to its durability, malleability, and aesthetic appeal. Nevertheless, it is common knowledge that the current supply of commercial timber raw materials needs to grow in quality and quantity. This creates a gap between the increase in demand and the decrease in the availability of wood raw materials. To solve this issue, it is necessary to utilize fast-growing timber species, one of which is samama wood (Cahyono et al., 2016).
Samama wood (Anthocephalus macrophyllus (Roxb) Havil) is the endemic wood from Sulawesi and Moluccas Islands, and it has begun to be extensively cultivated for industrial plantations (Cahyono et al., 2012). The annual growth rate in diameter of samama is between 5 and 7 cm. When the tree reaches maturity at the age of 10 years old, it may produce an average volume of 1.8 m3, a breast height diameter of up to 50 cm, and a branch-free height of 10–12 m (Lembaga Penelitian dan Pengembangan, 2011). In contrast, samama wood has a low density and strength, which is classified in the strength class of IV. The average specific gravity of samama wood is 0.47 (0.30–0.59). In addition, its average value of modulus of elasticity (MOE) and modulus of rupture (MOR) are 4,758.56 MPa and 59.40 MPa, respectively. These inferior properties impact its limited usage. Therefore, it is necessary to improve the quality of samama wood, especially its physical and mechanical properties to enhance its usage.
Wood impregnation is an approach for modifying wood by incorporating impregnating materials into the wood, preferably at the level of the wood cell walls. Wood impregnation has been extensively studied (Augustina et al., 2023a, 2023b; Dirna et al., 2020; Hadi et al., 2019, 2022; Laksono et al., 2023; Rahayu et al., 2019; Sumardi et al., 2020), but environmentally favorable impregnate materials, such as citric acid (CA), have not been widely utilized, particularly at high curing temperatures. CA (C6H8O7 = 2-hydroxy-1,2,3 propane-tricarboxylic acid) is a weak organic acid found in the leaves and fruit of citrus plants (oranges) and contains three carboxyl groups (Umemura et al., 2011). Additionally, Umemura et al. (2011) mentioned that CA is a natural preservative that can bind to hydroxyl groups in wood effectively, allowing it to be widely disseminated and create cross-links. A previous study by Rumbaremata et al. (2019) showed that the CA-compregnated wood achieved permanent fixation at 180°C of curing temperature for 1 hour. Therefore, in order to conceive the phenomenon during CA impregnation and enhance the dimensional stability and mechanical properties of CA-impregnated wood, the curing temperatures of 10% CA treatment were applied at 140°C, 160°C, and 180°C in this study (Table 1).
Treatments | Temperatures (°C) | Code |
---|---|---|
Untreated | - | C |
Citric acid-impregnated | 140 | CA 140 |
160 | CA 160 | |
180 | CA 180 | |
Heat-treated | 140 | HT 140 |
160 | HT 160 | |
180 | HT 180 |
Heat treatment has long been recognized as a potentially useful method to improve dimensional stabilization. The chemical composition of wood’s cell wall and extractives are altered during the heat treatment. The severity of this chemical composition alteration during the heating process depends on the temperature and duration of the treatment, with temperature being the most influential factor (Esteves and Pereira, 2009; Priadi et al., 2020). Heat treatment below 140°C results in only a slight change in the mechanical properties of wood. In contrast, the use of heating temperature above 140°C alters the wood’s physical and mechanical properties (Hill, 2006). At temperatures between 180°C and 260°C, the properties of wood change significantly, and above 260°C it will induce persistent degradation of wood components, then subsequently reduce the wood quality (Kocaefe et al., 2008). Therefore, appropriate heating temperature and duration are required for optimal results on heat treatment.
The objective of this study was to compare the dimensional stability and mechanical properties of samama wood that had been impregnated with CA at high curing temperatures with those of wood that had been heated at the same temperature. Anti-swelling efficiency (ASE) was used to detect the dimensional stability of treated wood. In addition, the weight percent gain (WPG) and weight loss (WL) were measured to observe the effect of impregnation and heat treatment on the dimensional stability of samama wood, respectively. Meanwhile, the MOE and MOR of untreated and treated samples were measured to observe the impact of both treatments on the mechanical properties of samama wood.
2. MATERIALS and METHODS
The sample used was samama wood (Anthocephallus macrophyllus (Roxb) Havil) with a diameter of ≈ 20–35 cm obtained from a well-managed plantation in Bogor, West Java, Indonesia. From the felled tree, a log section of 100 cm in length was taken from the bottom part of the tree stem. The sample log was sawn into a 3 cm thickness of board by a bandsaw. Boards samples were air-dried up to a moisture content of 12%–15% and sawn into smaller samples. Various physical properties, i.e., density, WPG, WL, and ASE, as well as mechanical properties, i.e., MOR and MOE was evaluated both in untreated and treated wood. The sample size of physical properties was 2 × 2 × 2 cm (longitudinal × tangential × radial), while that of mechanical properties was 30 × 2 × 2 cm. A schematic diagram of preparation samples and treatment is shown in Fig. 1.
The impregnation process was performed using a CA compound. In order to conceive the phenomenon during CA impregnation, different curing temperatures were applied at 140°C, 160°C, and 180°C. These treatments were compared with the heat treatment process at a similar temperature. Only the clear wood samples, namely free from knots, mold, fungi, and visible defects were tested. There were 105 samples of both untreated (15 replications) and treated wood (2 treatments × 15 replications × 3 temperatures) for each observed parameter.
Before proceeding to the next steps, all samples were measured to obtain their oven-dried volume and then weighed before treatment. The treatment was conducted in eight steps as follows: (1) putting the samples within CA solutions with 10% concentration (w/w) into a sealed impregnation chamber; (2) vacuum condition at –50 cmHg for 30 min; (3) pressure at 0.7 MPa for 3 h; (4) wrapped in aluminum foil for better polymerization; (5) heated for curing in an oven at 140°C, 160°C, and 180°C for 1 h; (6) oven-dried at 103 ± 2°C for 24 h; (7) conditioning overnight at 23°C and 80% ± 5% RH until constant weight was produced; (8) measured and weighed the samples after treatment.
A similar condition as above was performed on the samples before proceeding to the heat treatment process. The treatment was conducted in four steps as follows: (1) heat-treated in an oven at 140°C, 160°C, and 180°C for 1 h; (2) oven-dried at 103 ± 2°C for 24 h; (3) conditioning overnight at 23°C and 80% ± 5% RH until constant weight was produced; (4) measured and weighed the samples after treatment.
The untreated and treated samples for density measurement were prepared following the British Standard 373–1957 (BS, 1957) and calculated using the following Equation (1):
Where, W0 = the initial weight of wood in oven-dried condition (g), and V0 = the initial volume of wood in air-dried condition (cm3).
During those treatments, the samples could possibly gain some weight due to the impregnating agents penetrating the wood cells, and even lose some weight due to degradation of wood constituents. The percentage increase in weight (WPG) of the samples after impregnation and WL after heat treatment were calculated using the following Equations (2) and (3):
Where, W0 = weight of wood in oven-dried condition before treatment (g); Wt = weight of wood in oven-dried condition after impregnation process (g); Wht = weight of wood in oven-dried condition after heat treatment (g).
The dimensional stability of untreated and treated samples was approached by Rowell and Ellis (1978)’s method. The parameters consisted of coefficient of swelling (S) and ASE. Before proceeding to the process, all samples were measured and weighed to obtain their oven-dried volume and weight before treatment. Samples were immersed in distilled water for 24 h, ensuring that no sample floated on the water’s surface. Then, samples were dried at 103 ± 2°C. The sample dimensions were determined by length, width, and thickness at marked positions on the sample. One cycle of the soaking-drying system was conducted. The weight and volume of the samples were recorded after treatment. The S and ASE values after CA impregnation and heat treatment were measured according to Equations (4) and (5):
Where, V1 = volume of wood before immersion in water (cm3); V2 = volume of wood after immersion in water (cm3); S1 = volumetric swelling coefficient of untreated wood (%); S2 = volumetric swelling coefficient of treated wood (%).
The mechanical properties, i.e., elasticity and fracture toughness, of untreated, CA-impregnated, and heat treated were carried out by MOE and MOR tests using a Universal Testing Machine (UTM). The tests were performed according to the British Standard 373–1957 (BS, 1957). The samples were subjected to the three-point loading at a load of 50 kN right in the middle length of the samples with a pressure speed of 10 mm/minute. The maximum load (F-max) and the strain when the specimen breaks are recorded.
The MOR and MOE values of untreated and treated samples were measured according to Equations (6) and (7):
Where, Pmax = the maximal load when the sample is broken (N); ΔP = load difference in elasticity zone (N); L = the supporting span (mm); b = the width of the samples (mm); h = the thickness (depth) of the samples (mm); Δy = deflection at mid-length below the proportion deflection limit (mm).
The experimental design applied was randomized with two factors, namely treatment (three levels) and temperature (three levels). The data was presented as an average and standard deviation. Analysis of variance (ANOVA) was used to assess the effect of treatment given on density, WPG, WL, and dimensional stability (S and ASE) in untreated and treated wood. If there are significant differences between each factor and the interaction, it will be followed by Duncan’s multiple distance test.
3. RESULTS and DISCUSSION
The difference between the density, WPG, and WL values of each treatment, i.e., CA impregnation and heat treatment can be viewed in Figs. 2 and 3. It can be seen that interaction among treatments applied (CA impregnation and heat treatment) had a significant effect on density and WPG, while interaction among temperatures was not significantly affected (Table 2). Moreover, interaction among treatments and temperatures applied had a signification effect on WL (Table 2). The overall density (at 12% moisture content) of samama wood before treatment was on average 0.32 g.cm–3. Concerning each treatment applied, the highest density was found in the CA-impregnated samples and the lowest was found in the heat-treated samples. Regarding the temperature applied during the treatments, the highest density was found to be in the CA impregnated at 160°C and 180°C (0.36 g.cm–3), while the lowest was obtained in the heat-treated samples at 160°C and 180°C (0.31 g.cm–3).
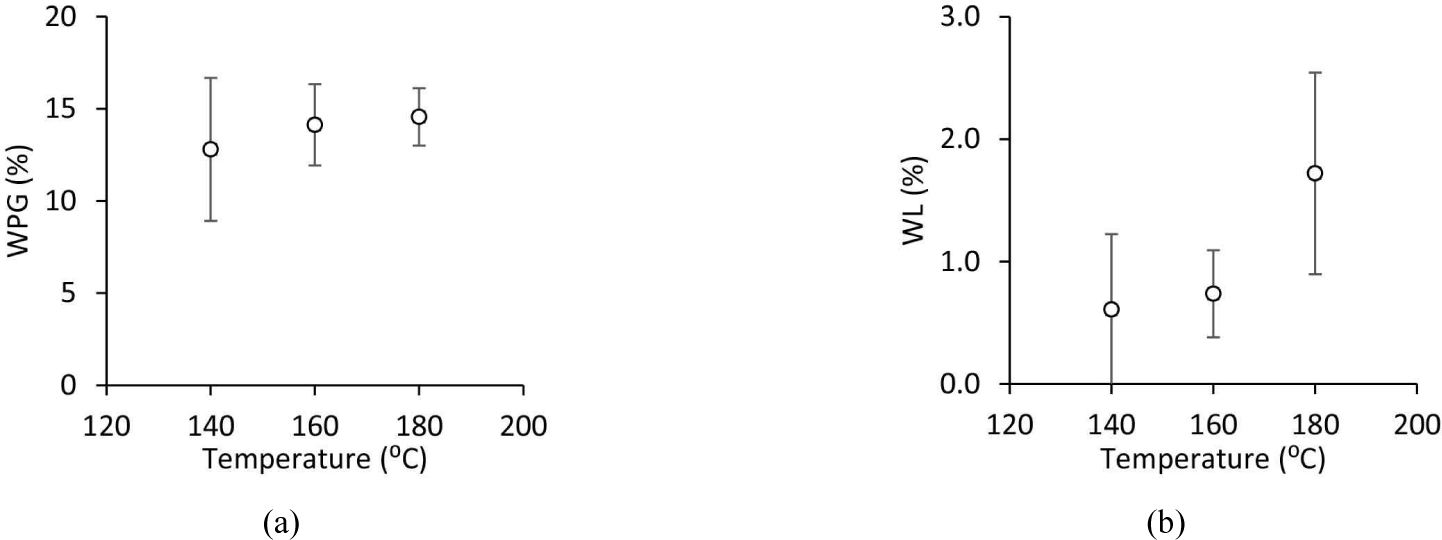
The increase in density for CA-impregnated is just slightly higher (by value) compared with other treatments. The values increased by 10.32% compared with untreated samples. This phenomenon was expected due to the penetration of the CA compound into the wood cells. This could be convinced by WPG values obtained after impregnation treatment, which was on average 13.82% [(Fig. 2(a)]. This value was relatively similar to Feng et al. (2014), around 15% of WPG obtained during 12% CA impregnation on poplar wood. In a previous study, it was explained that CA can act as cross-linking catalyst and/or cross-linking agent, and even a dispersing agent (Lee et al., 2020). In this case, CA mostly acted as a cross-linking agent between cell wall polymers by forming ester bonds/linkages. This phenomenon is in accordance with Augustina et al. (2023c), Feng et al. (2014), Laksono et al. (2023), and Lee et al. (2020). This mechanism tended to affect the density after impregnation treatments.
It also can be shown in Fig. 2, that a higher curing temperature applied in impregnation treatment gave higher density. This phenomenon is in accordance with the increase in WPG. Those values were 12.8%, 14.13%, and 14.56% in curing temperatures at 140°C, 160°C, and 180°C, respectively. It was found that both density and WPG values were higher at 160°C and 180°C treatments than those at 140°C treatments. These phenomena were in line with the research of Berube et al. (2018). It was found that crude glycerol and CA are cross-linked via the curing process at 160°C, then eventually forming an ester linkage and polymer network within wood cells. Katovic et al. (2004) added that the optimal crosslinking temperature for CA with cellulose is 180°C. This condition occurred because, when CA reacted with wood after impregnation, there were anhydride groups in CA that were released and bound to the hydroxyl groups of cellulose so that the anhydride bonds with these hydroxyl groups caused cross-linking (Mihulja et al., 2021).
On the other hand, heat treatment results in quite the opposite phenomenon than the impregnation process. This treatment caused a decrease in density values by 2.18% compared with untreated samples (Fig. 2). Increasing heat temperature during the process given a negative effect on density values. This can be explained by WL in wood cells during heat treatment. Heat treatment at 140°C, 160°C, and 180°C resulted in the loss of weight around 0.61%, 0.74%, and 1.72%, respectively. WL in the heat-treated wood occurred due to several factors. Heat treatment evaporated and removed water contained in the wood, both free and bound water (Esteves and Pereira, 2009; Priadi et al., 2023). These caused a decrease in weight because bound water was a relatively heavy component in wood. In addition, heat treatment also degraded chemical components in wood, such as hemicellulose, lignin, and amorphous parts of cellulose. Guller (2012) revealed that the decomposition and degradation reactions of chemical components were affected by temperature and duration of heat treatment. The heating treatment process breakdown of chemical bonds in these components, producing volatile compounds that were released from the wood, such as extractive substances, but new compounds could be formed due to the degradation of the structural components of the cell wall (Esteves and Pereira, 2009). These could also decrease the weight of the wood. In addition, factors that influence the WL were the heating medium (Esteves and Pereira, 2009), the density and chemical composition of wood (Chaouch et al., 2013), and wood species (Hill, 2007). The WL for hardwood species was reported to be higher than that of softwood species (Hill, 2007).
The combination of water removal and degradation of chemical components resulted in weight reduction in the heat-treated wood (Cahyono et al., 2022; Dos Santos et al., 2014; Kim et al., 2018; Schulz et al., 2021). This mechanism tended to affect the density after heat treatments. Furthermore, the reduction in weight was often considered an indication of the quality improvement for heat-treated samples, such as increasing the dimensional stability of the wood (Esteves and Pereira, 2009; Tuong and Li, 2010) or increasing resistance of wood-destroying insects/organisms.
Dimensional stability is one of the essential properties of wood, in which the dimensions of wood can alter in response to changes in ambient temperature and humidity. The dimensional stability of wood can be improved through heating and impregnation of CA, which is the thermal and chemical treatments. Both simultaneously have different effects on the ASE value of wood. Rautkari et al. (2014) reported that heating wood at temperatures between 150°C and 180°C increased the ASE value and flexural properties. In order to improve the dimensional stability, samama wood was simultaneously thermally and chemically modified in this investigation at distinct CA impregnation at curing temperatures of 140°C, 160°C, and 180°C.
Fig. 4 shows the ASE of samama wood after heat treatment (a) and CA impregnation (b). ASE values of heat-treated wood at 140°C, 160°C, and 180°C were 23.51%, 23.78%, and 22.21%, respectively. Meanwhile, ASE values of CA-impregnated wood at the same temperatures were 43.12%, 44.42%, and 35.37%, respectively. The average value of ASE for heat-treated samama wood was 23.17%, while those of impregnated samama wood were 40.97%. Fig. 4(a) demonstrates that heat treatment resulted in lower ASE values than CA impregnation because the volumetric swelling coefficients for the heat treatment were greater than those for CA impregnation after immersion in water. These facts indicated that there were still a significant number of free OH groups in wood that could be bonded to the water, causing the volume to expand. This result was in accordance with the finding of Karimi et al. (2013), who reported that expansion was caused by the formation of hydrogen bonds between the free OH group of hemicellulose and water by the absorption of water. Free OH group on hemicellulose could readily bind with water, resulting in a high swelling rate and low ASE value. The result showed the optimum ASE value was achieved at 160°C. It might be due to the reorientation of amorph cellulose, which improves dimensional stability. In contrast, it is shown that the ASE value of heat-treated wood decreased after heating at 180°C. It is caused by the degradation of cellulose. It was consistent with the finding of Lestari et al. (2021), who reported that cellulose could be degraded further in the heating of 180°C.
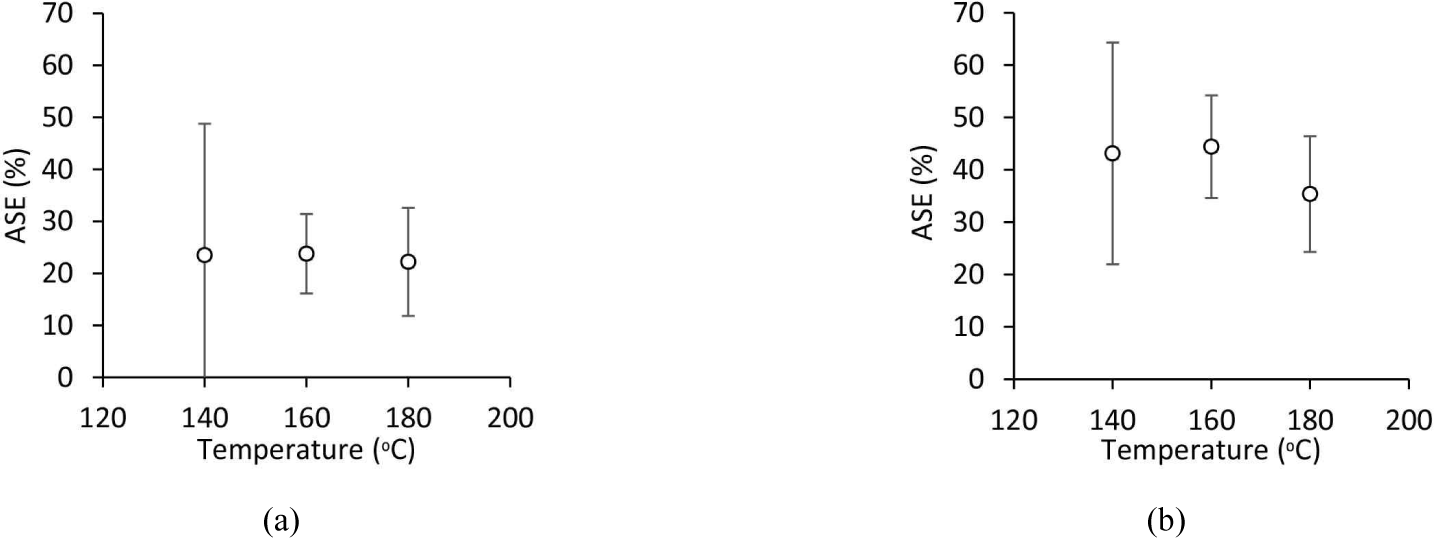
The effect of CA impregnation can be seen in Fig. 4(b). CA is an active constituent with three carboxyl groups that have been shown to be effective as a binder in wood compounds (Umemura et al., 2011). When samama wood was impregnated with CA, the free OH group in cellulose attached to the carboxyl group of CA, which replaced the OH group of water. As a result, it prevented the penetrating water into the wood cell cavities and cell walls. The formation bonding of the carboxyl group in CA and the hydroxyl group of wood causes a minor amount of expansion, thereby increasing the ASE value.
The result showed that the ASE value increased along with the increasing of curing temperature, which is only occurred up to the curing temperature of 160°C [Fig. 4(b)]. This was consistent with a study by Bekhta and Niemz (2003). They reported that wood becomes more stable with increasing heating temperature and duration. In addition, Kocaefe et al. (2008) reported that thermal treatment at 130°C could transform wood to be more hydrophobic. However, the ASE value of impregnated wood decreased at the curing temperature of 180°C. It could be due to the thermolysis process that happened during the curing at this temperature leading to the decrease in its properties.
The correlation between ASE and WL of heat-treated and WPG of CA-impregnated samama wood is depicted in Fig. 5. The ASE value of heat-treated wood ranged from 22.21%–23.78%, with the maximum ASE value recorded at 160°C. Meanwhile, the ASE values of CA-impregnated wood were in the range of 35.37%–44.42%, with the highest ASE value recorded in the WPG at 160°C.
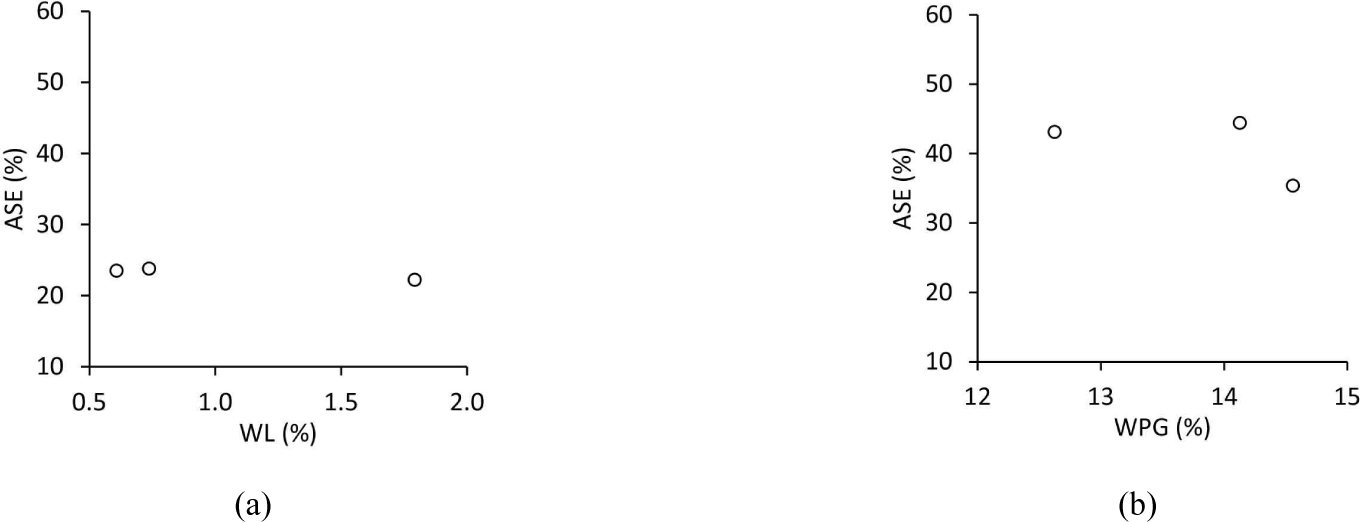
At low or moderate heat intensities, the reduction in wood weight tends to be positively correlated with ASE values [Fig. 5(a)]. As WL increases, so do the ASE values of heat-treated wood. The ability of wood to enlarge in high-humidity conditions has been successfully diminished by mild or moderate heat treatment. However, at high heat treatment intensities or severe degradation, extensive WL could destroy the cell wall integrity of the wood, resulting in a decrease in ASE values over time or at high temperatures.
Similarly, to the percentage of WL, the duration and temperature used affected the ASE values (Zhan et al., 2021). Moreover, Czajkowski et al. (2020) added that a decrease in ASE values could take place during prolonged exposure to elevated temperatures. With an increase in heat treatment time or temperature, the macromolecular components of the cell wall suffered chemical alterations, which were accompanied by a decrease in weight (Hill, 2007). Chemical degradation correlates positively with ASE values when the heat treatment intensity was low or moderate (Esteves and Pereira, 2009). However, extensive degradation could disrupt the integrity of the cell wall (Czajkowski et al., 2020), resulting in a decrease in ASE values when exposed to high temperatures or for an extended period. This study determined that the optimal thermal treatment temperature for increasing the dimensional stability of samama wood is 160°C.
Fig. 5(b) demonstrates that the optimal curing temperature for CA impregnation also stands at 160°C. Cahyono et al. (2020) studied the impregnation of Methyl Meta Acrylate (MMA) to samama wood. They reported that there is a decrease in the weight of impregnated wood at a curing temperature of 180°C, after experiencing a significant weight increment at a curing temperature of 90°C. In addition, Bourgois et al. (1989) added that the higher the temperature, the higher the severity of wood degradation wood, with the WL reaching 30% after 30 minutes at 260°C. Loss of timber weight is an indicator of the quality of modified wood, according to Esteves and Pereira (2009). During heat treatment, hemicellulose was predominantly degraded, klason lignin increased, and wood acidity decreased (Awoyemi and Westermark, 2005). The degradation of these chemical components is determined by the treatment’s duration and temperature (Esteves and Pereira, 2009).
Mechanical properties tests were carried out to determine MOE and MOR values. The MOE or elasticity strength is a measure of the wood’s resistance to maintain its shape in relation to the wood’s stiffness. MOE occurs due to loading and is only valid up to the proportion limit. The higher the MOE value of wood, the more resistant it is to deformation. Thus, the ability to withstand loads is greater (Wulandari et al., 2020). Meanwhile, MOR or fracture toughness is the wood’s ability to withstand loads up to the maximum limit (Wulandari et al., 2020).
The mechanical properties of samama wood under different treatments are described in Table 3. Average values of MOE and MOR were 4,758.56 MPa and 59.40 MPa, 4,778.28 MPa, and 39.62 MPa, as well as 4,608.53 MPa and 53.07 MPa for untreated, CA-impregnated and heat-treated, respectively. It can be seen that interaction among temperatures applied (CA impregnation and heat treatment) has a significant effect on MOE, while interaction among treatments was not significantly affected (Table 2). Moreover, the interaction among treatments and temperatures applied have a signification effect on MOR (Table 2). Compared with untreated samples, the average MOE values tended to increase by 0.41% after CA impregnation, while the opposite phenomenon occurred after heat treatment. The slight increase in MOE value for CA impregnation was expected due to the presence of the impregnation compound within wood cells. It is convinced by the increase in WPG values during the impregnation treatment [Fig. 6(a)]. This compound could fill the void spaces and form ester linkage with wood polymer, then eventually affect their mechanical properties, especially MOE value. A similar phenomenon was reported by Feng et al. (2014). On the other hand, the average MOE values for heat treatment decreased by 3.15% than that of untreated samples. This can be explained by the degradation of the wood polymer, mostly hemicellulose and partly cellulose, as well as the changes in substance. It was convinced by WL after treatment [Fig. 6(b)]. Moreover, the average bending strength (MOR) values were decreased by 31.35% and 8.05% for CA-impregnated and heat-treated, respectively. Higher decrease of MOR values after CA treatment due to a combined effect of temperature and acid, which caused severe loss of mechanical properties. According to Feng et al. (2014), CA treatment results in the reduction of MOR values by ca. 20%–30% irrespective of the WPG level. In addition, it was found that CA treatment could make the wood become more brittle, then considerably lose its impact strength of ca. 50% (Feng et al., 2014).
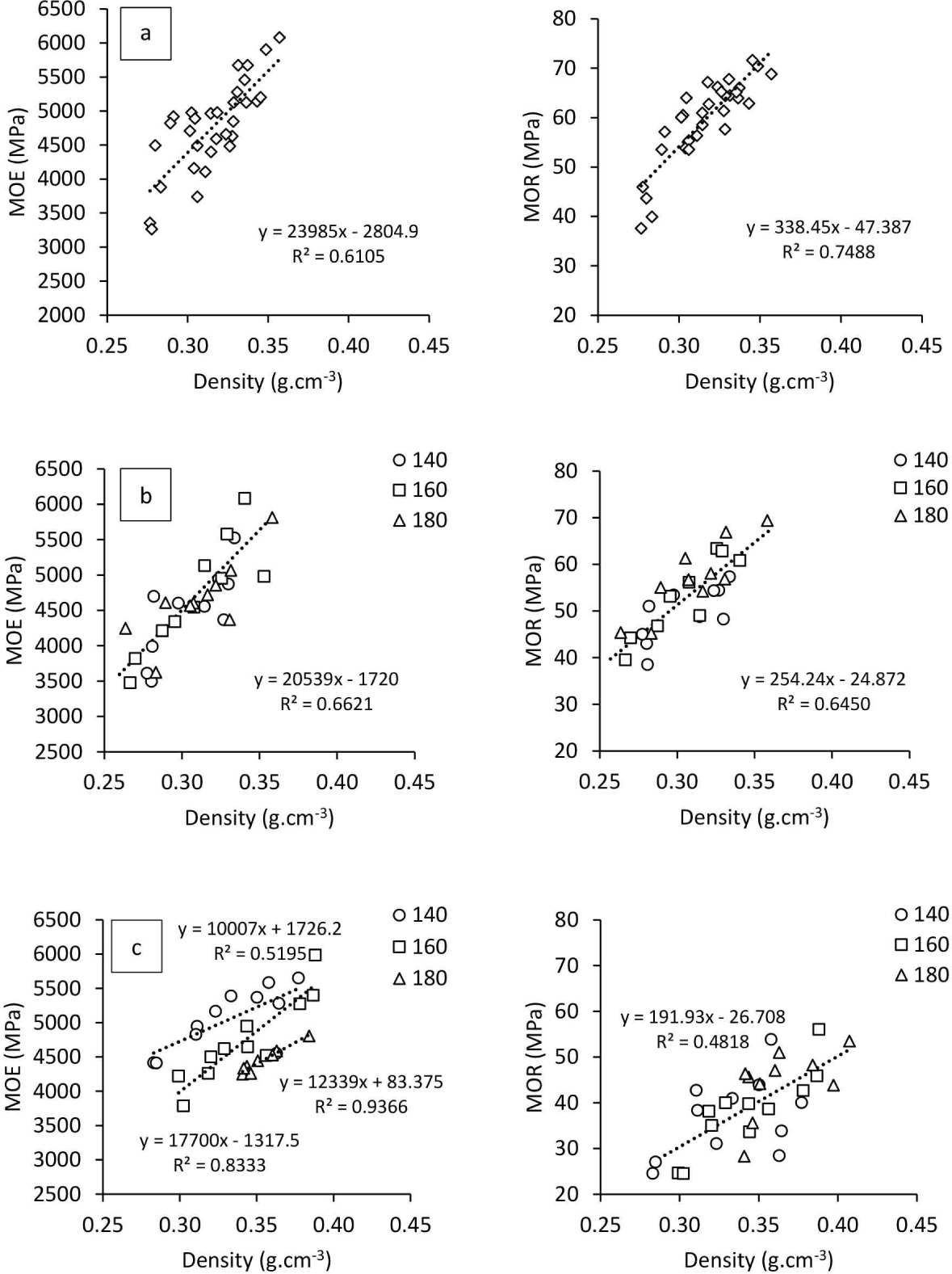
Even though the overall values decreased compared with untreated samples, especially MOR values, hence it also did vary between temperatures applied. The MOE value for CA-impregnated samples is higher than that of untreated at 140°C, then eventually started to decrease with the increase of temperature (160°C and 180°C). As stated before, the CA compound is cross-linked via curing at 160°C and started to form ester linkage during the process. The presence of this compound with the combination of heat (temperature) results in thermolysis decomposition which is elevated the degradation within wood polymers. A similar phenomenon was reported by, Siregar (2011), Widsten et al. (2014) and Widyorini et al. (2015), decomposition and acid degradation of polysaccharides increased with the increase of curing temperature. It also noted that the thermolysis decomposition of CA started at > 161°C (Widsten et al., 2014). Furthermore, the MOE values for heat-treated samples increased during 140°C to 160°C treatment, followed by a small decrease after 180°C treatment. A similar phenomenon was reported by Peng et al. (2022), the MOE changes by 3%, 1%, and –5% at 120°C, 160°C, and 200°C, respectively.
Meanwhile, the MOR values tended to increase as the temperature increased in both treatments (Table 3). This could be due short duration of treatment applied which caused less temperature exposure. This phenomenon is in accordance with Peng et al. (2022). It can be found that during heat treatment at 120°C, MOR tends to increase in the first 16 h and then decreases with increasing time; at 160°C treatment, those value increases up to 5 h, then decrease remarkably at longer duration; at 200°C treatment, a steady decrease occurs since the first few hours caused the extent degradation. A similar case was also reported by Poncsák et al. (2006) that stated the MOR values decrease along with the increase in temperature, particularly above 200°C. This was associated with the breakdown of hemicellulose and cellulose, as well as the formation of crystalized cellulose within the wood polymers. Peng et al. (2022) convinced this phenomenon by stated short duration with high-temperature treatment tended to have similar phenomena to the lower temperature treatment, while other phenomena occurred when the duration of treatment was extended.
That phenomenon could also be viewed from the relationships between MOE and MOR with density for untreated [Fig. 6(a)], heat-treated [Fig. 6(b)], and CA-impregnated [Fig. 6(c)] samama wood. The density in each treatment has a moderate to high correlation with their mechanical properties. The relationships between the density of untreated wood with MOE and MOR values were expressed by linear regressions y = 23,985x – 2,804.9, R2 = 0.61; and y = 338.45x – 47.387, R2 = 0.75, respectively. Those values tended to change after both treatments were applied, thus affecting the slope of regression (Fig. 6). The relationship between those values for heat treatment was expressed by y = 20,539x – 1,720, R2 = 0.66; and y = 254.24x – 24.872, R2 = 0.65, respectively. The slope of regression for this treatment tends to be more dispersed and no trend occurred within the temperature applied. Moreover, the slope of CA treatment tends to be more aligned with each temperature applied, especially, the MOE values. The relationship between density and MOE values for CA treatment at 140°C, 160°C, and 180°C of curing temperature were expressed by y = 10,007x + 1,726.2, R2 = 0.52; y = 17,700x – 1,317.5, R2 = 0.83, and y = 12,339x + 83.375, R2 = 0.94, respectively. While, for MOR values were expressed by y = 191.93x – 26.708, R2 = 0.48. The correlation between those values is generally dependent on the species and treatment applied. Those treatments certainly could affect the wood properties, in some cases might be attributed to the changes in microfibril angle/ MFA, the degradation, and or changes in the wood polymers.
Based on these findings, the changes of MOE (by values) are less than MOR in both treatments. A similar phenomenon was also reported by Peng et al. (2022). The overall mechanical properties after CA are much better than that of heat treatment, except for MOR values. The optimum temperature is suggested at 160°C in both treatments.
4. CONCLUSIONS
The wood modification through CA impregnation and heat treatment at different curing and heat temperature, i.e., 140°C, 160°C, and 180°C, were performed over physical and mechanical properties. Concerning the treatments applied, the highest density was found in the CA-impregnated samples, while the lowest was found in the heat-treated samples at 160°C and 180°C, respectively. The increase in temperature during CA impregnation was directly proportional to WPG, while heat treatment was directly proportional to WL. Higher temperatures applied during both treatments were directly proportional to the dimensional stability (up to 160°C), but inversely proportional to the mechanical properties, except for MOR. CA impregnation (ASE of ca. 40%) had higher dimensional stability than heat treatment (ASE of ca. 20%) at a similar condition applied. The MOE for CA-impregnated samples is higher at 140°C, then eventually started to decrease with the increase of temperature; while for heat-treated samples, the values increased during 140°C to 160°C treatment, followed by a small decrease after 180°C. Meanwhile, the MOR values tended to increase as the temperature increased in both treatments, but the values are lower than that of untreated. CA impregnated had higher density, dimensional stability, and mechanical properties, except for MOR, than that of heat treatment. The optimum temperature is suggested at 160°C in both treatments.