1. INTRODUCTION
Noise pollution is regarded as one of the most threatening environmental pollutions to humankind, second only to air and water pollution (Patel et al., 2021). Long-term exposure to noise pollution can lead to chronic diseases such as hearing damage, stress, sleep disturbances, high blood pressure, and heart problems (Yang, 2020).
Since the COVID-19 pandemic, the time spent indoors has increased. As the use of online education, telecommunication, and video conferencing increases, the demand for improving the acoustic environment at home or in the office also increases (Andargie et al., 2021). The number of households raising dogs in apartments also increases exponentially in Korea (Park et al., 2021) and family dogs make indoor noise at times and cause disputes between neighbors (O’Brien, 2020).
To overcome noise pollution and associated problems, sound-absorbing materials such as urethane foam, synthetic fiber, and mineral fiber have been introduced. These sound-absorbing materials have been widely used as they are cost-effective, easy to mold, and demonstrate excellent sound-absorbing performance (Arenas and Crocker, 2010).
Recycling these commercially available materials is, however, difficult and may adversely affect human health. In particular, studies have shown an increased risk of respiratory cancer among workers who produce or discard mineral fiber (Gualtieri, 2021; Metintas et al., 2019). In addition, employees exposed to styrene may have a cancer risk in the petrochemical industry (Moshiran et al., 2021).
Various types of natural fibrous materials derived mostly from agricultural industry have been researched as an alternative to commercially available sound-absorbing materials. These materials are, for example, coir fiber (Nor et al., 2010), kenaf fiber (Taban et al., 2020), and kapok fiber (Xiang et al., 2013), and rice by-products (Kang et al., 2019b). Natural fibrous materials exhibit a sound absorption coefficient (the frequency range between 200 and 2,000 Hz) comparable to that of commercially available sound-absorbing materials (Iannace, 2017).
Research has demonstrated that the highest value of the sound absorption coefficient has shifted to the lower frequency as the thickness of the experimented materials has increased. The sound absorption coefficient value also increases as the thickness of the materials increases (Kang et al., 2019b; Taban et al., 2020).
Wood is familiar and sustainable resources as a building material from prehistoric times to the present (Ghani and Lee, 2021; Hadi et al., 2022; Jamaludin et al., 2020; Kim and Kim, 2020). Wood has been also widely researched as a sound absorbing material. Plywood can be used as a resonance type sound absorber by adjusting the hole size and air back cavity (Peng et al., 2018). Cross-sections of hardwoods also can be used as a porous sound absorber and their high through pore porosity is found to be advantageous for sound absorption (Jang and Kang, 2021a, 2021b, 2021c; Jang and Kang, 2022).
Wood bark particles can be used as a granular type sound absorber (Kang et al., 2019a; Tudor et al., 2020) while evergreen tree leaves used as sound-absorbing materials (Jung et al., 2020). Wood pellets also has sound absorption effect (Jang, 2022). This previous study indicates that not only solid wood but also its by-products can be used as sound-absorbing materials.
Pine (Pinus densiflora) pollen has various nutrients and physiologically active ingredients, widely used as food and herbal medicine (Hou et al., 2017). However, the pine pollen cone was known to be a useless by-product. This study focused on using pine pollen cones as a sound-absorbing material. The pine pollen cone has a low density, its granules can absorb noise using their hollow space, and their rough surfaces can scatter sound waves. This study investigated the sound absorption properties of pine pollen cones using impedance tubes. This study is the new approach that proposes pine pollen cones as an alternative for an eco-friendly sound-absorbing material.
2. MATERIALS and METHODS
Pine pollen cone samples shown in Fig. 1 were collected in the front yard of College of Education at Jeonbuk National University (Jeonju, Korea). These pine pollen cone samples had an abscissa length of approximately 0.8–1.2 cm and an ordinate length of approximately 3.5–4.5 cm.
One pine pollen cone sample was dried in a laboratory oven at 40°C for 5 hours. The sample was then loaded into an ion coater (SCM, Emcrafts, Korea) and coated with gold. The SEM (Genesis-1000, Emcrafts, Korea) was used to examine a sample at 100× and 500× magnification in high-vacuum mode (7.5 × 10–5 mmHg).
Fig. 2 shows the schematic diagram of the impedance tubes (type 4206, Brüel & Kjaer, Denmark) to measure the sound absorption coefficient. The impedance tubes consist of a large impedance tube (99 mm diameter) and a small (29 mm diameter) tube. The pine pollens with a height of 6 to 12 cm were loaded to large and small impedance tubes. Their apparent density in the impedance tubes was kept at 0.085 g/cm3.
A large impedance tube was used to measure the absorption coefficient in the frequency range of 100–1,600 Hz, while a small impedance tube was used to evaluate the absorption coefficient in the frequency range of 500–6,400 Hz. The range of the sound absorption coefficient is from 0 to 1.
Environmental condition during measurement of the sound absorption coefficient was as follows; an atmospheric pressure was 1,018.00 hPa, a temperature was 10.10°C, a relative humidity was 31.50%, the velocity of sound was 337.39 m/s, the density of air was 1.251 kg/m3, and the characteristic impedance of air was 421.7 Pa/(m/s).
3. RESULTS and DISCUSSION
Fig. 3(a) shows SEM images of the pine pollen cones at 100 magnification, and Fig. 3(b) and Fig. 3(c) show SEM images at 500 magnification. The pine pollen cone has a form of overlapping broad, thin scale-like shapes, and curved and rough surfaces. When the incident sound waves encounter these structures, they can be diffracted or scattered, and advantageous for sound absorption.
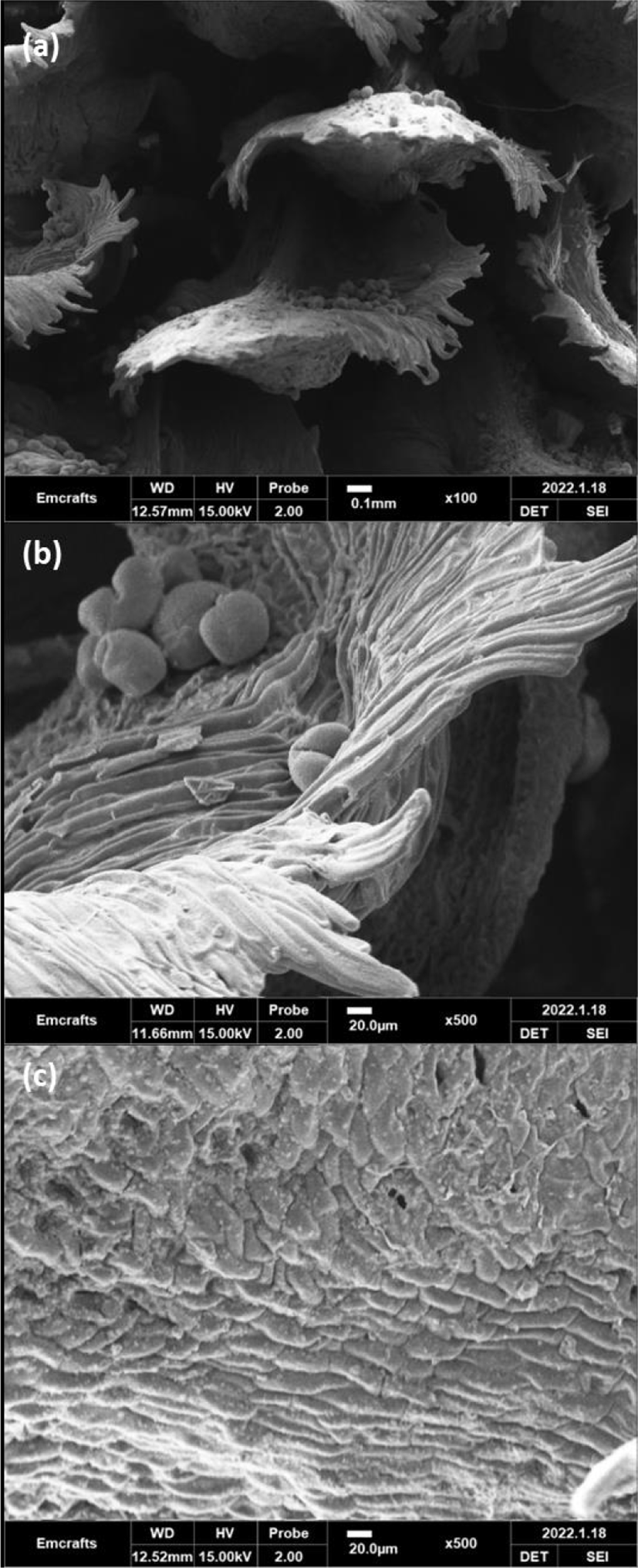
Fig. 4 shows the frequency of pine pollen cones’ acoustic absorption curves. Fig. 4(a) demonstrates the results from the large impedance tubes, and Fig. 4(b) the results from the small impedance tubes.
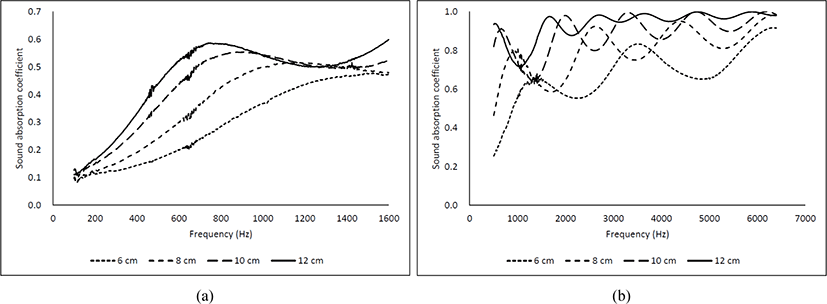
As the thickness of the pine pollen cones filled in the impedance tubes increased, the sound absorption coefficient at low frequencies also increased. The maximum sound absorption coefficient at 6 cm thickness was 0.477 at 1,512 Hz, 0.515 at 1,122 Hz for 8 cm thickness, and 0.555 at 910 Hz for 10 cm thickness, 0.586 at 740 Hz for 12 cm thickness [Fig. 4(a)].
At high frequencies above 1,000 Hz, the number of sound absorption peaks increased as the thickness of the pine pollen cones increased. At 6 cm thickness, 3 sound absorption peaks appeared, 8 cm showed 4, 10 cm demonstrated 5, and 12 cm identified 6 peaks. For every 2 cm thickness increases, the sound absorption peak increased by 1. At a thickness of 12 cm, the sound absorption coefficient was close to unity above approximately 1,500 Hz. Pine pollen cones are, therefore, should be considered a very useful material for high-frequency sound absorption.
The dominant frequency range of family dog barks is between 160 and 2,630 Hz (Pongrácz et al., 2010). The results of this study identified 12 cm thickness pine pollens used as a sound-absorbing material can cover a significant portion of this frequency range, which may help reduce barking noise.
Table 1 summarizes the sound absorption coefficients (250, 500, 1,000, and 2,000 Hz) and the NRC (Noise Reduction Coefficient). When the thickness was doubled from 6 cm to 12 cm, the sound absorption coefficient increased by 1.7 times at 250 Hz, 2.6 times at 500 Hz, 1.5 times at 1,000 Hz, and 1.6 times at 2,000 Hz. As a result, the NRC was 1.7 times increased.
Kang et al. (2019a) reported the sound absorption performance of sound absorbers made of wood bark particles. Their NRC (10 cm thickness) was 0.24 for Radiata pine, 0.30 for Hemlock, 0.30 for Elm, 0.31 for Douglas-fir, 0.57 for Larch, and 0.80 for Hinoki. The sound absorption properties of the pine pollen cones at the same thickness were equivalent to or higher than that of the wood particles. This is thought to be because the surface of pine pollen is rough, and many scale-like curves facilitate sound wave scattering.
The Korean Industrial Standard KS F 3503 (KSA, 2012) classifies the sound-absorbing properties into four grades (0.3 M grade: 0.21–0.40, 0.5 M grade: 0.41–0.60, 0.7 M grade: 0.61–0.80, and 0.9 M grade: above 0.81) depending on the NRC of the sound absorption materials. This study found that pine pollen cones had a sound absorption grade of 0.3 M (NRC: 0.305) in 6 cm, 0.3 M (0.481) in 8 cm, and 0.5 M (NRC: 0.513) in 10 cm, and 0.5 M (0.517) in 12 cm.
The sound-absorbing performance of pine pollen cones was sufficient to be considered an alternative to commercially available synthetic sound-absorbing materials. Pine pollen cones once were a useless resource for which no one cared. However, this study demonstrated that pine pollen cones have utility value as an eco-friendly sound-absorbing material.
4. CONCLUSIONS
The sound absorption properties of pine pollen cones were investigated in this work. As the thickness of pine pollen cones increases, the sound absorption ability improves. Pine pollen cones had a sound absorption grade of 0.3 M to 0.5 M (KS F 3503) depending on their filling thickness. Pine pollen is a valuable resource widely used as food and herbal medicine, but pine cones were a wasted resource. Conclusively, this study proposes using pine pollen cones as an environmentally friendly sound-absorbing material.