1. INTRODUCTION
Enzymatic CO2 conversion has been researched as a potential method to greenhouse gas fixation and the production of renewable chemicals (Crable et al., 2011). Formate dehydrogenases (FDHs) are oxidoreductases that catalyzes the conversion of formate into CO2; they have been extensively used for cofactor recycling in chemoenzymatic processes (Barin et al., 2019). Recently, several FDHs from bacteria, yeast, and plants have been shown to possess activity in the reverse reaction, that is, the mineralization of CO2 into formate (Yu et al., 2019). FDHs can be classified into three categories, metal independent/NAD(nicotinamide adenine dinucleotide)(P)+-dependent FDHs, metal-containing/NAD(P)+ independent FDH, and metal-containing/NAD(P)+-dependent FDH (Maia et al., 2015). Although NAD-dependent FDHs have limited by drawbacks such as high cost and instability of reduced form of NAD (NADH), FDH (CbFDH) isolated from Candida boidinii is commercially available and has been adopted as a CO2-reducing biocatalyst in enzymatic reaction systems (Calzadiaz-Ramirez and Meyer, 2022). However, the CO2-reducing activity of CbFDH is still very low for practical application (Choe et al., 2014).
In this study, we research CO2-reducing performance of wood rot fungus, T. versicolor. White rot fungi, a type of basidiomycetes, have been known as a major cause of wood biodegradation (Ham et al., 2021). They have also especially acid-producing abilities; oxalic acid and formic acid are the major acidic metabolites (Shimada et al., 1997). FDH is known as an enzyme that induces an oxidation of formate, a metabolite of wood rot fungi, can be decomposed into CO2 (Watanabe et al., 2008). In the case of wood-rot fungi, studies have mainly focused on ligninolytic enzyme system for the lignin degradation (Hong et al., 2013; Kim and Bae, 2007; Lee et al., 2005). Laccase of ligninolytic enzymes has been shown effective in various biotechnological processes such as pulping and bleaching and bioremediation of environmental pollutants (Choi et al., 2006; Jung, 2019; Park et al., 2020). Also, enzyme related to secondary metabolism was reported in previous research (An et al., 2018). However, the reduction activity of FDH from wood-rotting fungi has not been characterized. Thus, we were motivated to characterize FDH from the white-rot fungus T. versicolor.
2. MATERIALS and METHODS
Commercial enzymes and high-pressure liquid chromatography (HPLC) standards were purchased from Sigma-Aldrich (Merk KGaA, Darmstadt, Germany). Expression vector (pBIVT) and AccuRapidTM protein expression kit were purchased from Bioneer (Daejeon, Korea).
Fungal culture was isolated from fruitbody collected by the National Institute of Forest Science (Seoul, Korea) in 2020. The fungal fruitbody was identified as T. versicolor based on morphological characteristics. The T. versicolor sample was inoculated on potato dextrose agar (PDA) with, 10 ppm of benomyl and 100 ppm of streptomycin sulfate, and cultured at room temperature.
The fungal isolate was identified based on internal transcribed spacer (ITS) sequences. Genomic DNA was extracted from the fungal cultures using AccuPrep genomic DNA extraction kit. The primers for polymerase chain reaction (PCR) were ITS5 (5′-GGA AGT AAA AGT CGT AAC AAG G-3′) and ITS4 (5′-TCC TCC GCT TAT TGA TAT GC-3′). PCR amplification conditions followed temperature cycling parameters; 95°C for 5 min, followed by 30 cycles of 95°C for 30 s, 55°C for 30 s, and 72°C for 60 s. An elongation step of 72°C for 5 min was performed at the end. The obtained ITS sequence was proofread and identified using Nucleotide BLAST (www.ncbi.nlm.nih.gov). Our ITS sequence was identified with the type specimen of T. versicolor (GenBank Acc. No.: NR_154494), showing high similarity (99.6%).
The mycelia cultured on PDA medium were scraped and homogenized in sterile distilled water. The homogenized liquid containing the mycelium was oven-dried and inoculated into 100 mL of Potato Dextrose Broth medium so that the oven dried mycelia reached a concentration of 0.1%. Then, formic acid (5 and 10 mM) was added into an aqueous medium. The medium was shake-cultured at 100 rpm and 25°C for 6, 9, or 12 days. The formic acid concentration was calculated by HPLC (1290 Infinity II, Agilent Technologies, Santa Clara, CA, USA). AccuPower reverse-transcription polymerase chain reaction (RT-PCR) Premix (Bioneer) was used to detect the expression level of mRNA encoding FDH. The reaction mixture was analyzed by agarose gel electrophoresis. Gene-specific PCR primers were as follows: F: 5′-TGT ACT ATG CTC ACT TCC ACC A-3′ and R: 5′-CTC ATC CCA CGG TTA CAG G-3′.
The TvFDH gene was amplified by PCR using T. versicolor cDNA with primers (F: 5′-TGT ACT ATG CTC ACT TCC ACC AGG ATC C-3′ and R: 5′-CTC ATC CCA CGG TTA CAG GCG CCG GCG-3′). The PCR-amplified DNA was gel-purified and sub-cloned into pCR2.1-Topo vector. And then, the open reading frame of TvFDH was digested with BamHI and NotI, and ligated using T4 DNA ligase, and was inserted into pBIVT vector.
The expression of recombinant TvFDH protein was conducted using the Accu RapidTM cell-free protein expression kit (Bioneer). Cell-free protein synthesis is a valuable tool for understanding how mRNAs are translated into functional polypeptides (Katzen et al., 2005). The sample of recombinant TvFDH was concentrated using a centrifugal device according to the instructions suggested by the manufacturer. The expressed proteins were then visualized by electrophoresis on a sodium dodecyl sulphate-polyacrylamide gel electrophoresis (SDS-PAGE) followed by Coomassie Brilliant Blue R-250 staining.
The FDH activity was determined by monitoring the absorbance change at 340 nm during the redox reaction catalyzed by cell-free recombinant proteins. The oxidation of formic acid was conducted using an assay solution (2 mL of a 100 mM sodium phosphate buffer at pH 7) containing 20 μL of cell-free proteins, 10 mM formic acid, and 2 mM NAD+. The reduction of CO2 was conducted using an assay solution (2 mL of a 100 mM sodium phosphate buffer at pH 7) containing 20 μL of recombinant protein, 50 mM NaHCO3, and 0.15 mM NADH. In the case of the CO2 reduction reaction, NaHCO3 was used as a substrate to supply CO2 because the concentration of gaseous CO2 cannot be accurately determined.
The amount of formic acid produced was determined by HPLC (1290 Infinity II, Agilent Technologies) with a refractive index detector (RID). The column was an Aminex HPX-87H (300 × 7.8 mm, Bio-Lad Laboratories, Hercules, CA, USA). Sulfuric acid solution (0.01 N) was used as the mobile phase with a flow of 0.6 mL/ min.
3. RESULTS and DISCUSSION
In our previous study, mycelium growth of 60 species under formic acid were identified to screen for biocatalysts from wood rot fungi. Of the 60 specimens collected, T. versicolor showed the highest growth rate (Jang et al., 2021).
To evaluate the oxidation activity of the T. versicolor whole cell, formic acid was added into mycelium cultures as a substrate. After 144 h of incubation, formic acid had decreased by more than 90% in the culture of T. versicolor treated with 5 mM formic acid. In cultures with 10 mM formic acid, substrate concentration was decreased by 7.7% after 72 h and 68.7% after 216 h. These results indicate that T. versicolor has the formic acid oxidation activity (Fig. 1b).
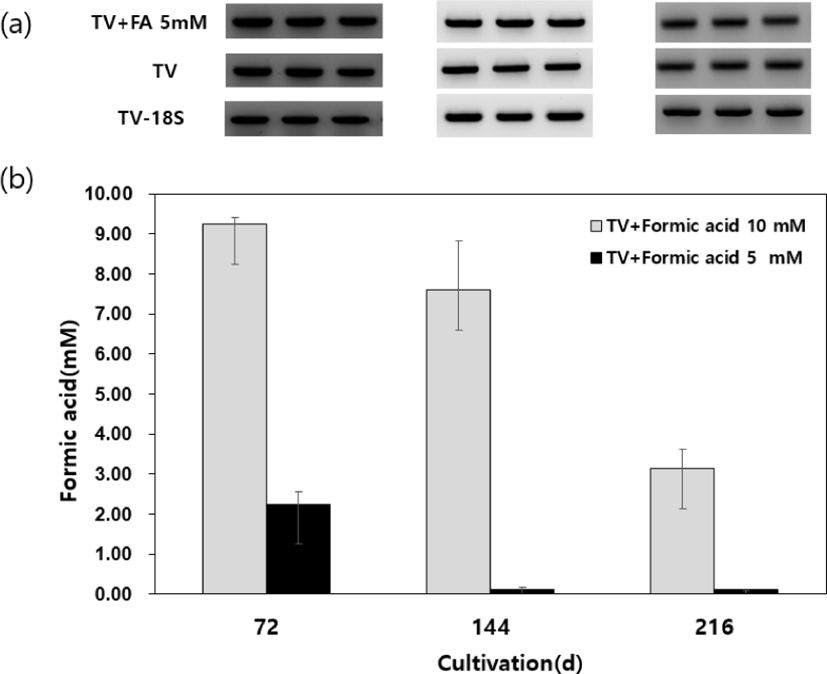
Gene expression of FDH gene was confirmed from the strain cultured at the same time by reverse transcription-polymerase chain reaction (RT-PCR). However, significant change in expression level was not observed by 5 mM formic acid (Fig. 1a). We assumed that FDH plays an essential biochemical role in T. versicolor during vegetative growth. According to the prior studies, FDH play an important role in decomposing oxalate to reduce NADH as an energy source during the vegetative growth of white-rot fungus Ceriporiopsis subvermispora (Watanabe et al., 2008). Wood rot fungi have been shown to produce NAD-dependent FDH as an intracellular enzyme, which decomposes formate, the reaction product of oxalate by oxalate decarboxylase, resulting in the formation of CO2 (Mäkelä et al., 2014). However, the reduction activity of FDH from wood-rotting fungi has not been characterized.
The open reading frame (ORF) of TvFDH consisted of 1,200 bp nucleotides encoding 399 amino acid. The deduced protein sequence of TvFDH showed similarity with other wood rot fungi such as Trametes sp., Lenzites betulinus, Pilatotrama ljubarskyi etc. The BLAST search analysis for TvFDH revealed 56% identities with well-characterized FDH from the yeast Candida boidinii (GenBank accession no. AF004094). From the conserved domain architecture retrieval tool (CDART) analysis, NAD-binding domain were found in TvFDH at amino acid positions 174–234, 195, 256, 282, and 311–314 (Fig. 2). In CbFDH, conserved three-glycine pattern (G/A)XGXXG plays an important role in providing the specificity to NAD+ versus NADP+ (Schirwitz et al., 2007). This conserved motif was also identified in the TvFDH (Table 1). However, NAD binding site of TvFDH at position 233 was predicted differently than CbFDH. The roles depending on amino acid will be investigated using the purified proteins.
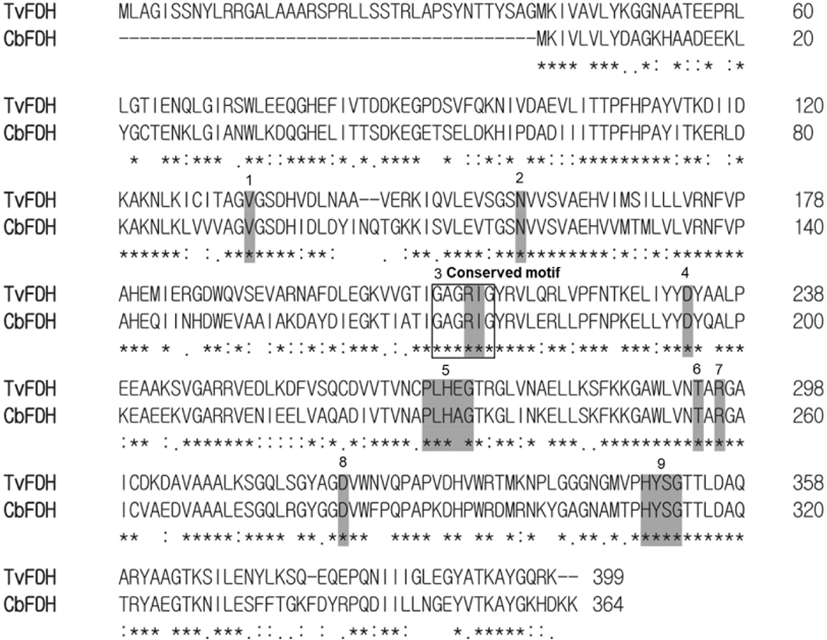
TvFDH ORF of the pCR2.1-Topo clone was separated and was transferred to a Cell-free expression vector, pBIVT vector tagged six-histidine (Fig. 3a). The recombinant gene in the plasmid was verified by PCR. Three recombinants (T-6, T-7, and T-8) of digested plasmids were obtained and showed gene expression at the expected size (Fig. 3b). These results show that the TvFDH gene had been successfully inserted into the pBIVT-N-His plasmid. The plasmids T-6, T-7, and T-8 were followed by the in vitro expression using a cell-free protein expression kit. The expression of the recombinant proteins of samples (T-6, T-7, and T-8) was visualized via SDS-page electrophoresis. The SDS-PAGE gel exhibited molecular mass (42 kDa) of the samples that were slightly over-expressed compared with the controls (Fig. 3c).
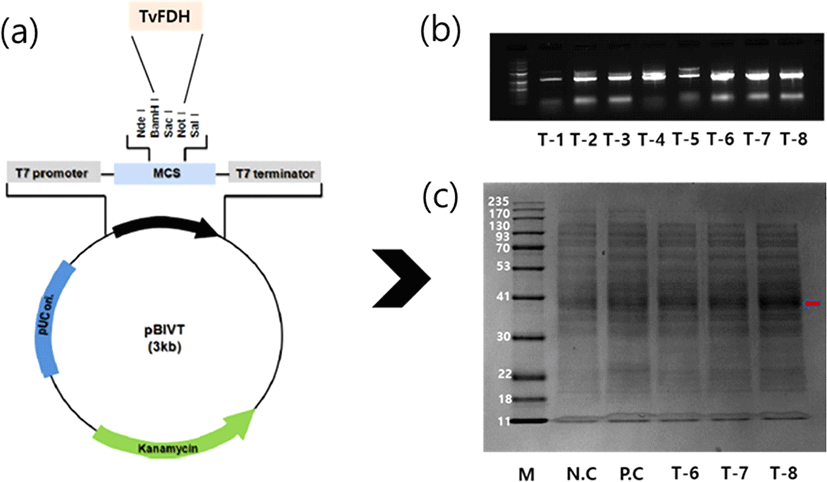
As shown in Fig. 4, the sample of T-8 has the highest enzyme activity in oxidation and reduction reaction. The NADH measurement at 340 nm was increased from 0.10 to 0.33 (Fig. 4). In the reduction reaction, the NADH absorbance by T-8 was decreased from 0.39 to 0.29 (Fig. 5). After measuring enzyme activities, the products of samples (T-6 to T-8) were analyzed by HPLC. As the results, cell-free recombinant proteins produced 0.09–0.17 mM of formic acid after 60 min (Table 2). These results of enzyme activities and metabolites analysis showed that the recombinant TvFDH proteins were expressed by a cell-free protein expression system.
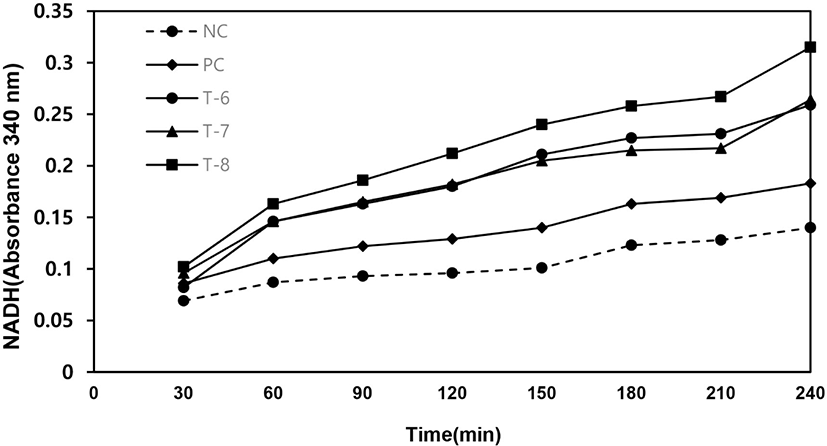
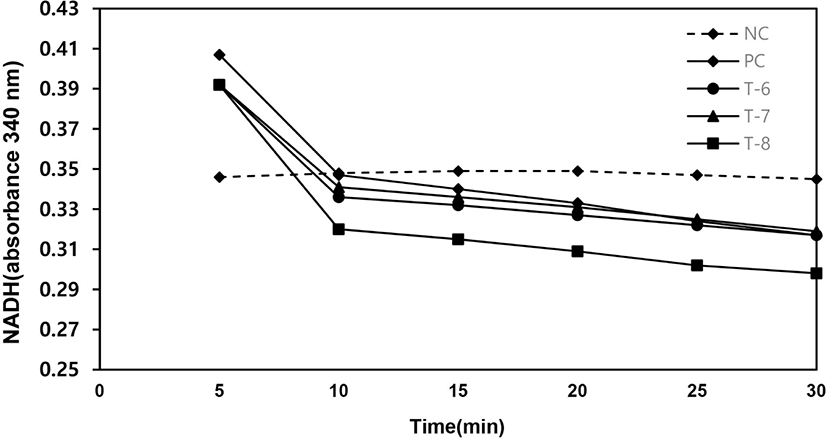
NC | PC | T-6 | T-7 | T-8 | |
---|---|---|---|---|---|
Reduction (mM) | ND | ND | 0.09 | 0.12 | 0.17 |
FDHs are produced by bacteria, fungi, and plants, but few species of wood rot fungi have been characterized from C. subvermispora (Watanabe et al., 2005), and Dichomitus squalenes (Mäkelä et al., 2014). FDHs have been suggested to participate in the breakdown of oxalate metabolite by wood rot fungi (Shimada et al., 1994). More recently, FDH have been proposed to have a broader physiological role than detoxification. It has been suggested to participate in energy production using NADH inside the fungal cells (Mäkelä et al., 2014).
Reduction activity of FDH enzyme from white rot fungi has not been characterized in this study. We suggest CO2 reduction by TvFDH isolated from T. versicolor based the previous suggested biological function of FDH.
4. CONCLUSIONS
Enzymatic conversion for CO2 utilization offers a green and promising approach to reduce global warming and climate changes. In this study, we successfully cloned full-length TvFDH and expressed it in a cell-free protein expression system. We characterized the cell-free extract with NADH reduction and oxidation activity. TvFDH is a candidate enzyme for industrial applications of CO2 conversion.