1. INTRODUCTION
Indonesia is well known to have a very high level of biodiversity, especially in terms of its vegetation diversity. One of the provinces with high biodiversity is North Sumatra. It has a tropical forest area with a relatively high level of species diversity and with several local vegetation, which may be studied for their characteristics. The forest area is around 3,010,160.89 ha or 41.25% of the total land area of North Sumatra Province. Several plant species are local commodities of North Sumatra, namely Kemenyan (Styrax sumatrana, Styrax benzoin Dryan, Styrax benzoine var. Hiliferum), Dryobalanop aromatica, Raru (Cotylelobium melanoxylon, Cotylelobium lanceolatum, Vatica pauciflora), etc. The characteristics of some local species have not been studied.
Several studies were conducted by Iswanto et al. (2016) regarding the physical and mechanical properties of three species of Styrax wood originating from North Tapanuli, and the chemical composition based on the differences in the growth location of Styrax sumatrana was also studied. A study was conducted on the potential of styrax resin as an antioxidant and the cinnamic acid content found in the styrax resin of North Tapanuli (Hidayat et al., 2018; Kiswandono et al., 2019). In other related studies, Susilowati et al. (2018) and Rahmat et al. (2017) detailed the genetics of styrax grown in North Sumatra, Indonesia. It was reported that the styrax plant can be used for other purposes, such as medicine, perfume, cosmetics, etc.
The Raru plants are the other plant species that can be further studied in the forest areas of North Sumatra. Based on the available information, particularly of the Central Tapanuli area, there are three species of Raru producing plants (Susilowati et al., 2020; Elfiati et al., 2019). They include Dahanon (Cotylelobium lanceolatum), Songal (Vatica pauciflora), and Pulut (Cotylelobium melanoxylon). Hildebrand (1954) showed that there are several species of wood classified as raru producing plants, including Shorea maxwelliana King, Vatica songa V.Sl. from the Dipterocarpaceae, and Garciniasp. from the Guttifera family.
Generally, the bark of raru wood is used to improve the taste and the alcohol content of Bataknese traditional toddy beverage (Susilowati et al., 2019), antihyperuricemic, and antioxidant (Sinaga et al., 2020). The wood is suitable for heavy material constructions, such as electricity poles, and in ship-building (Susilowati et al., 2020). Furthermore, it can be used as natural food preservatives due to its high tannin content (Pasaribu, 2007). However, there is limited information related to the basic properties of this wood. The data found were still limited in terms of the physical, mechanical, and chemical properties of Raru C. Melanoxylon as reported by Pasaribu (2007) and Pasaribu et al. (2007).
As an effort to optimize the use of the wood, information related to the characteristics is required. Several basic properties have an important role in the use of wood as raw material. One of which is the chemical component consisting of cellulose, hemicellulose, lignin, and extractives. Henriksson et al. (2009) reported that the chemical properties, which include cellulose, extractive, and lignin content, are some of the properties that have an impact on pulp and paper production. Furthermore, the chemical properties are also important and should be considered because they are associated with several uses. In the form of cellulose content, these properties can be a reference for using wood as a bioethanol material (Demirbas, 2005). The chemical properties serve as a reference for the use of wood as a pulp and paper material (Sjöström, 1993). The chemical properties in lignin and cellulose content affect the heating value of wood (Bowyer et al., 2003; Lee et al., 2019). Furthermore, the properties can be considered for any purpose such as the cellulose for capacitor material (Li et al., 2019) to replace the use of ink on plastics with colored films (Tzeng et al., 2015), and as a raw material for 3D printing ink (Mietner et al., 2021). The lignin can be utilized for raw material in wastewater treatment (Xiao et al., 2019; Maulina et al., 2020; Fatriasari et al., 2020), biomedicine (Siddiqui et al., 2018), biorefinery (Liu et al., 2019; Yang et al., 2019), paint and coating (Zikeli et al., 2019), and packaging (Xing et al., 2019).
This study focused on examining the effect of wood species and the axial position of the stem on its chemical components. These two factors play an important role in determining the diversity of chemical components of wood. The variation of the chemical properties was affected by species differences and log positions. In addition, (González-Rodrigo et al., 2013) reported that phenotypic and genotypic factors cause a high variability in wood properties between different and individuals of the same species. Individual variations are not apparent but resulted from a complex system of various factors involved in modifying the physiological processes of wood formation. Previous studies showed that there are various properties such as a chemical component of lignocellulosic materials including wood. Meanwhile, Lestari et al. (2016) reported that the extractive content of platinum teak wood is significantly affected by tree age and axial position in the stem. Pettersen (1984) further claimed that chemical component variations are influenced by wood section (roots, stems, and branches), species, growing location, climate, and soil conditions. The chemical makeup differs from pith to bark, from stump to crown, from earlywood and latewood, and between sapwood and heartwood in each portion of a single tree (Kollmann and Cote, 1984). In addition, genetic factors and tree age also have an impact on the chemical composition of wood (Berrocal et al., 2004). Therefore, an axial study of the chemical properties of Raru wood based on the log positions was conducted. This study aimed to analyze the chemical composition of three species of Raru wood (C. lanceolatum, C. melanoxylon, and V. pauciflora) originating from Central Tapanuli, North Sumatra, Indonesia based on the axial positions of the logs.
2. MATERIALS and METHODS
Raru wood species of C. lanceolatum, V. pauciflora, and C. melanoxylon were collected from Central Tapanuli Regency, North Sumatra Province (1° 11' 00"- 2° 22' 0" north latitude, and 98° 07'- 98° 12' east longitude). They were C. lanceolatum, V. pauciflora, and C. melanoxylon with the criteria of a tree diameter greater than 15 cm. In addition, only one tree was used for each species and was converted into three log sections after been felled. Fig. 1 showed the base, middle, and top of the wood.
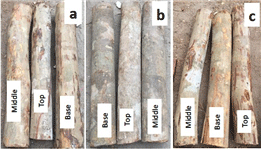
The samples were then ground with a ring flaker for over 40 mesh, then oven-dried with a maximum temperature of 40°C. They were then stored in sealed plastic containers for further testing, as shown in Fig. 2. The preparation was based on the Indonesia National Standard (SNI 08-7070: 2005). For chemical component analysis, the moisture content was determined using an oven which was set at a temperature of 105 ± 3°C for 24 h, and it ranged from 2-4% for lignin analysis, 3-6% for ethanol and benzene, and 1-2% for alpha-cellulose.
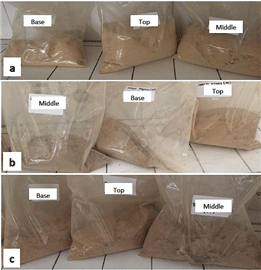
The chemical component analysis was performed based on the Indonesian National Standard (SNI). This standard was used to determine the chemical components of wood (Hastuti et al., 2017; Purwita and Sugesty, 2018; Jasni et al., 2016). The analysis was observed following the structural chemical compositions of the cell wall, such as Acid-insoluble lignin (SNI 8429: 2017), alpha-cellulose (SNI 8400: 2017), and holocellulose (ASTM D1104–56). Furthermore, extractives content as a non-structural chemical component was also observed. The extractive in alcohol benzene was performed based on SNI 8401:2017, and its solubility in cold and hot water, as well as 1% NaOH, was referred to the SNI 01-1305-1989 and SNI 14-1838-1990.
In the 50 mL cup, about 1.0 ± 0.1g free ethanol benzene extracted samples were placed, and 15 mL of 72% sulfuric acid was added and stirred for 2-3 min until fully dispersed. Furthermore, 300 mL of distilled water was added to a 1000 mL Erlenmeyer, and water was applied until the amount reached 575 mL, at which point the sulfuric acid concentration reached 3%. The solution was heated and the temperature was maintained for 4 h with a constant volume. Meanwhile, the deposit was separated and washed with hot water until acid-free, then precipitated in an oven at 105 ± 3°C.
A 2 g sample of the ethanol-benzene extracted was weighted and washed with hot water before the benzene alcohol solution was removed. It was wetted with cold water (10°C) and placed in a filtering paper containing the sample in the chlorination apparatus and chlorinated for 3 min. The filtering paper was then carefully stirred, and the chlorination was repeated for 2 min before being washed with 95% alcohol to dissolve the excess chlorine gas and HCl. After 1 min, a vacuum pump was used to remove the solution and moistened with cold water. In addition, menoethanolamine 3% (± 75°C) was added and left for 2 min before removing the solution with a vacuum pump. This step was repeated once more, and the residue was washed twice with 95% alcohol and twice with cold water. The solvent was extracted from each washing by using a vacuum pump, and the chlorination was repeated every 2-3 min, followed by two washes with 95% alcohol to extract the alcohol-monoethanol solution. The residue was washed two more times with cold water and 95% alcohol until it was no longer acidic. Furthermore, ether was added to speed up the drying process, and the residue-filled filtering paper was oven dried for 2.5 h at 105 ± 3°C, then cooled in the desiccator and weighed.
The Alpha-Cellulose content was determined by weighing 1.5 ± 0.1 g and placing it in a 300 mL cup glass with 75 mL of 17.5% NaOH solution, which was heated in a 25 ± 0.2°C until the sample was dispersed. To make a 100 mL complete solution, 25 mL of 17.5% NaOH solution was added. After 30 min of the first NaOH solution addition, 100 mL of aquades was added and left in the hot plate stirrer for 30 min until the total extraction time is approximately 60 min. After that, 25 mL of the filtrate and 10 mL of 0.5 N potassium dichromate solution were put into a 250 mL flask, then 50 mL of concentrated H2SO4 was added. The solution was left for 15 min in the hot plate stirrer. Furthermore, 50 mL of aquades was added and left to cool, and 2-4 drops of pheromoin indicator were titrated with 0.1 N ferro ammonium sulfate solution until purple. The filtrate was replaced with 12.5 mL of 17.5% NaOH solution and 12.5 mL of aquades for the blank titration.
About 1 ± 0.1 g of sample powder (40-60 mesh) was weighed in a petri dish, and then samples were put in the filtering paper, covered and tied. The soluble fraction in ethanol benzene of samples was then calculated. Following that, the samples were put in a 250 mL soxhlet apparatus (the initial weight was determined), and 150-200 mL of a 1:2 alcohol-benzene mixture was applied. This apparatus was connected to the cooler and extracted in a water bath for 6 h, with five circulations per hour. Furthermore, the filtering paper containing samples was removed from the apparatus, and the extraction solution was steamed until nearly dry. The residual evaporation was heated in pumpkin extraction at 105°C for 3 h or more.
A total of 2.0 ± 0.1 g of oven-dried samples were inserted into the 200 mL Erlenmeyer flask and 100 mL water was added before placing the cooler upright. In addition, the sample-containing Erlenmeyer was placed in a water bath containing boiling water for 3 h, with the surface in the bath set to be higher than that in the Erlenmeyer. The sample was filtered with a mouthpiece filtering paper considered to weigh after Erlenmeyer was removed from the water bath. Furthermore, the filtrate was then washed with hot water until it was clear, and the residue was then dried in a 105 ± 3°C oven for 4 h before being cooled in a desiccator and measured.
A total of 2.0 ± 0.1 g of oven-dried samples was inserted into a 400 mL cup glass and added 300 mL of water. The solution was stirred and placed at a room temperature of 23 ± 2°C for 48 h. Also, the sample was filtered with a masir funnel and washed with cold water until the filtrate was clear. The funnel containing the sample residue was oven-dried at a temperature of 105 ± 3°C for 4 h then cooled in a desiccator and weighed.
2.0± 0.1 g of oven-dried sample was transferred into a 200 mL cup glass, and 100 mL of 1% NaOH solution was added and stirred in a closed glass cup. For 60 min, it was put in a water bath containing boiling water and positioned where water in the handler was higher than the surface of the NaOH solution in the cup glass. The sample was filtered with a mouthpiece filtering paper that was known to weigh after the cup glass containing the sample was removed from the water bath. Furthermore, it was washed with hot water until the filtrate was colorless. 25 mL of 10% acetic acid was applied and allowed to stand for 1 min before re-filtration, and the items used were washed in hot water until they were acid-free. The glass containing the sample was removed from the water bath, and the sample was filtered with a mouthpiece filtering paper used for weighing. The funnel containing the sample residue was dried at 105 ± 3°C for 4 h before being cooled in a desiccator and weighed.
A completely factorial randomized design consisting of factors A and B was used. Factor A includes the three species of wood (C. lanceolatum,V. pauciflora, and C. melanoxylon), and factor B includes the three positions of the stem in the axial direction (base, middle, and top). The sample analysis for the chemical component was carried out in duplo for each treatment. Therefore, the total sample analyzed was 18 units. Analysis of Variance (ANOVA) test at a 95% confidence interval was conducted to determine whether this treatment provided a real difference or not to the chemical component parameters tested.
3. Results and Discussion
The average value of structural chemical composition was presented in Table 1.
The lignin content based on the axial direction ranges from 22.77 (1.09) - 31.25 (0.21)%, and the highest was located in the middle part of V. pauciflora wood, while the lowest was found in the top part of C. melanoxylon wood. The highest and lowest lignin content of all wood species was located in the middle and top part of the log. This result was contrary to a previous study conducted by Rahman et al. (2018) concerning lignin content in Kelampayan wood. It was reported that the highest lignin content was located in the top position because it was dominated by juvenile wood. Furthermore, raru wood was not significantly different concerning specific gravity at the base (1.07), middle (0.97), and top (0.93). The three positions are included in the category of high specific gravity since the phenomenon of the influence of juvenile wood on kelampayan as proposed by Rahman et al. (2018) does not occur in this raru wood.
Pasaribu (2007) showed that the specific gravity of C. melanoxylon wood at the three log’s position of the base, middle, and top trunk ranged from 1.02 to 1.09. The highest and lowest value was located in the base and then the top respectively.
All parts of V. pauciflora contain lignin, which showed that the wood has better strength than the other species. Lignin contributes to the stiffness of cell walls (Gindl et al., 2002), and Fagerstedt et al. (2015) stated that it provides resistance to the comprehensive force of structures.
Referring to the classification introduced by the Ministry of Environment and Forestry (2020), the lignin content was classified into the moderate category. Therefore, the three wood species can still be used as raw materials for pulp and paper. According to Martawijaya et al. (2005), the lignin content in wood commonly ranges from 18-33%, and a high content is not desirable in wood utilization for pulp and paper and bioenergy. This is because it needs to increase the pulp quality or accessibility of enzymes into cellulose. Lignin is the second-largest substance and one of the main chemical components in plants (Casey, 1980). It functions as a binding agent between cells and provides rigidity Ray et al. (2009). According to Terashima et al. (2009), lignin plays an essential role in the structural assembly of a cell wall.
The lignin content of these three wood species is almost the same as that of Acacia mangium, which ranges from 28-32% (Siagian et al., 2009), Shorea retusa,Shorea macroptera, and Eucalyptus pelita contents range from 26-31%, 24-31% (Yunanta et al., 2014; Lukmandaru et al., 2016), and 22-36% (Fatimah et al., 2013) respectively. They have a higher content when compared to Styrax sumatrana, Ceiba pentandra, and Ochroma pyramidale (Iswanto et al., 2019; Purnamawati et al., 2018). When compared with several subtropical wood species such as Larch, Red pine, Korean pine, Cypress, and Cedar as reported by Park et al. (2017), the lignin content of the three species of Raru wood is almost similar.
The analysis of variance (ANOVA) showed that the interaction between wood species and log position does not significantly affect the 95% confidence interval on lignin content. However, for a single wood species factor, it has a significantly different effect.
The holocellulose content of the three observed wood species with an axial direction of log ranged from 59.59 (0.83) to 69.00 (0.85)%. Meanwhile, the highest holocellulose content was found in the top part of V. pauciflora wood, and the lowest was seen in the bottom part of C. melanoxylon. According to Fengel and Wegener (1984), a small percentage of lignin remains in holocellulose. This is because there is an ultrastructural and chemical relationship between cell wall macromolecules, such as holocellulose and lignin.
This is slightly different from the result obtained from the study conducted by Rahman et al. (2018). It was reported that kelampayan wood, one of the fastest-growing species, had a holocellulose content ranging from 68 to 70%. Most of the cells are dominated by juvenile wood, which has a higher cellulose content. Furthermore, high cellulose affects the high holocellulose content, and the three Raru wood species were not classified as fast-growing wood due to their high specific gravity value.
Based on the species variety, C. melanoxylon wood has the highest holocellulose content in all positions. Therefore, it is predicted to have a higher pulp yield than V. pauciflora and C. lanceolatum. Most of the three Raru wood species contain high holocellulose content (> 65%), which produces good pulp characteristics and a high yield pulp (Fatriasari and Hermiati, 2008). A high proportion of polysaccharides showed a high holocellulose content, which will increase the pulp yield (MacLeod, 2007).
The analysis of variance (ANOVA) showed that the interaction of wood species with log position has a significantly different effect at a 95% confidence interval on holocellulose content.
Material with a higher alpha-cellulose content has high-quality raw material (Sumada et al., 2011). The cellulose content of Raru wood ranges from 40.46 (1.50) - 44.99 (1.12)%. This is consistent with Pettersen (1984), stating that the cellulose content of dry wood weight ranges from 40-50%. Generally, the wood growth environment contributes to variation of wood’s chemical composition, including cellulose (Fatriasari et al., 2019).
The higher content of cellulose is found in the middle position. The highest and the lowest alpha-cellulose contents are located in the middle and the top position of C. melanoxylon wood. Meanwhile, the purity is often expressed in the alpha-cellulose content. A high cellulose content showed strong fiber, relatively resistant in separation and purification to chemicals. It is also insoluble in neutral organic solvents and water (Casey, 1980). High alpha-cellulose such as cellulose nitrite, carboxyl methylcellulose, and cellulose xanthate is needed in making filter paper (Fengel and Wegener, 1984).
The three Raru wood species have almost similar cellulose contents and are presumably affected by the high specific gravity category (> 0.8). They have almost the same proportion of mature wood since the cellulose content does not differ. Furthermore, Ona et al. (1998) reported that the specific gravity of wood is positively correlated with cellulose content. The Indonesian wood classification assessment for pulp and paper material Ministry of Environment and Forestry (2020) showed that the cellulose content of Raru wood can be categorized into medium class.
The analysis of variance (ANOVA) showed that the interaction of wood species with log position has a significantly different effect at a 95% confidence interval on alpha-cellulose content.
The hemicellulose content of the three Raru wood species ranges from 19.13 (0.18) - 25.71 (0.44)%. Meanwhile, the highest and the lowest hemicellulose contents were found on the top log of V. pauciflora and C. melanoxylon respectively. This value was slightly lower than the hemicellulose content of Styrax sumatrana wood. Iswanto et al. (2019) also reported that Styrax sumatrana wood has a hemicellulose content ranging from 26-29%. Syafii and Siregar (2006) reported that a hemicellulose content with good criteria for pulp and paper raw materials ranges from 15 - 25%. A high hemicellulose content has an essential role in milling the pulp (Stephenson, 1951). It creates more flexible fiber, and it is critical to the fibrillation process. Besides, the fiber would be more plastic because of higher water absorption. This condition causes a high surface area formation during pulp formation Fatriasari and Hermiati (2008). This is due to the gelatin properties of hemicellulose, which facilitates the hydrophilic character of the pulp and facilitates the formation of bonds between fibers.
The analysis of variance (ANOVA) showed that the interaction of wood species with log position has a significantly different effect at a 95% confidence interval on hemicellulose content.
The average value of non-structural chemical composition was presented in Table 2.
The extractive substances are dissolved in both polar and non-polar solvents such as an alcohol-benzene solution of 1: 2. C. melanoxylon had the highest value in alcohol-benzene of 1:2 on the base, middle, and top positions with 14.18 (0.25)%, 14.92 (0.88)%, and 14.51 (0.58)% respectively. These values increased up to 5%, which can be included in high solubilization. In addition, it is less suitable to form good pulp properties (Fatriasari et al., 2015) based on the assessment of Indonesian wood classification for pulp and paper material (Ministry of Environment and Forestry, 2020). The extractive solubility in alcohol-benzene of 1:2 also significantly influences the wood color. Lukmandaru (2016) showed a higher extractive content in alcohol-benzene produces lower brightness of wood, which means the heartwood will be darker. C. melanoxylon wood has a higher extractive content and produces the darkest color compared to others. On the contrary, this high value in C. melanoxylon wood showed it is more difficult to be converted for pulp. This value needs a high amount of chemicals for the cooking process in the pulping process (Fatriasari and Hermiati, 2008).
The analysis of variance (ANOVA) showed that the interaction of wood species with log position has a significantly different effect at a 95% confidence interval on alcohol benzene 1:2 content.
Pari et al. (2006); Fengel and Wegener (1984) reported that tannins, gums, sugar, and coloring agents can be dissolved in the extraction using hot and cold water, while starches can only be solubilized by hot water.
Extractive solubility in hot and cold water ranged from 6.57 (0.66) to 8.15 (0.21)% and 2.48 (0.54) to 6.05 (0.11)% respectively. The highest and the lowest values in hot water were found in C. melanoxylon and C. lanceolatum wood respectively. Furthermore, the axial direction showed that the top and the base positions of C. melanoxylon Raru wood log have the highest and the lowest extractive content values dissolved in cold water. Generally, there was no difference in extractive content values based on the axial position of the log since hot water is a more solubilize extractive substance than cold water. The partial hydrolysis of hemicellulose that involves the development of an organic acid solution into the water may cause it (Dockzekalska et al., 2010). Also, starch solubilization in hot water extraction contributes to the higher values of the content. This is caused by partial hydrolysis of hemicellulose, which involves developing an organic acid solution into water.
The analysis of variance (ANOVA) showed that the interaction between wood species and log position does not significantly affect the 95% confidence interval. However, the log position has a significantly different effect on the extractive content that dissolves in hot water. For the cold water, the analysis of variance (ANOVA) showed that the interaction of wood species with log position has a significantly different effect at a 95% confidence interval on extractive content that dissolves in cold water.
The extractives solubility in 1%-NaOH ranged from 26.87 (0.95) to 29.76 (0.65)%. In the base position of C. melanoxylon wood, the solubility was higher than the others. Furthermore, the 1%-NaOH solubility showed the dissolving of wood components in low molecular weight of carbohydrates, tannin, and dyes. The high extractive substances in 1%-NaOH solvent indicated that the wood was dominated by inorganic minerals, carbohydrates, fats, oils, dyes, and aromatic compounds (Pari et al., 2006). In pulping process, the extractive substances are expected to be low due to decreased chemical consumption. A high extractive content should be able to produce pitch in the paper sheet.
The analysis of variance (ANOVA) showed that the interaction of wood species with log position has a significantly different effect at a 95% confidence interval on extractive content that dissolves in NaOH.
4. CONCLUSION
-
The mean values of chemical components (α-cellulose, hemicellulose, and lignin) for C. lanceolatum species were 41.88%, 19.39%, and 28.68%, respectively. For C. Melanoxylon and V. pauciflora the values were (42.01%, 21.11%, 24.76%) and (42.95%, 23.24%, 30.11%) respectively. Furthermore, the average values of the extractive contents (the solubility in 1:2 ethanol benzene, NaOH, and hot water) for C. lanceolatum,C. melanoxylon, and V. pauciflora wood were (10.58%, 27.62%, 8.13%), (14.54%, 28.22%, 7.82%), and (10.95%, 28.60%, 7.57%) respectively.
-
Wood species significantly affect the chemical components such as lignin, cellulose, and hemicellulose, and extractive solubility in cold water. Furthermore, the axial log position has a significant effect on the chemical component tested.
-
V. pauciflora wood had the highest cellulose, hemicellulose, and lignin content compared to the other species. The highest extractives solubility in alcohol-benzene, cold and hot water were found in C. melanoxylon wood. Meanwhile, the fractionation of structural component of high chemical in raru woods brings more wide range future application such as biorefinery products.