1. INTRODUCTION
Essential oils are secondary plant metabolites mainly composed of monoterpenes and sesquiterpenes (Mahmoud et al., 2002; Min et al., 2017). Essential oils have been of interest worldwide for their application in alternative medicines to treat diseases and for their stress-relieving, anti-inflammatory, anti-oxidant, and anti-microbial properties (Pujiarti et al., 2016; Elshafie et al., 2017; Yang et al., 2019; Ham et al., 2020; Lee et al., 2021). Whereas, at the same time, researchers have indicated that essential oils need further research (Farrar et al., 2020). Systematic and rigorous approaches to studying the biological activities of potential phytotherapeutics have only been initiated in recent decades (Ahn et al., 2018; Ahn et al., 2020). Phytotherapeutics such as essential oils are natural substances, but natural should not be considered synonymous with harmless. Evaluation of the toxicity of essential oils and regulation of their use are underway. The toxicity of essential oil may be considered trivial compared to that of conventional medicines; however, such essential oils could bring forth severe public health problems as the occurrence of side effects of essential oils is common. Moreover, there is possible toxicity resulting from improper use. Toxicity tests are designed to assess or predict toxic effects in vivo and to assess the relative toxicity of substances. In vitro cytotoxicity assaying is the first approach to evaluating the biocompatibility of an essential oil (Rogero et al., 2003).
Natural products such as essential oils can also interact with DNA, producing changes in its structure or function, and such modifications can trigger the cell replication system (Edris, 2007). A cell replication cycle is a series of events within a cell as it grows and divides. A cell spends most of its time in what is called interphase, and during this time, it grows, replicates its chromosomes, and prepares for cell division (Baserga, 1965). The G1/S and G2/M cell-cycle checkpoints maintain genomic stability in eukaryotes in response to genotoxic stress (Wang et al., 1999).
Cinnamomum loureirii is from the plant family Lauraceae, which has a worldwide distribution in tropical and warm climates. Many Lauraceae contain high concentrations of essential oils, some of which are valued for use in spices and perfumes (Prabuseenivasan et al., 2006). Within Lauraceae plants, most such substances are components of their irritant or toxic saps or tissues, which may repel or poison many herbivorous or parasitic organisms (Schausberger, 2018). Juniperus chinensis L. and Juniperus chinensis L. var. sargentii Henry is from the plant family Cupressaceae. The Cupressaceae may be a rich source of anti‐tick compounds, and further investigation of cypress species for repellent and insecticidal chemicals is warranted (Dolan et al., 2007; Dolan et al., 2009). Aster glehnii F. Schmidt and Artemisia capillaris Thunb. are from the plant family Asteraceae, which includes more than 1,600 genera and 20,000 species of herbs and trees worldwide. Zanthoxylum schinifolium Siebold & Zucc, Zanthoxylum piperitum (L.) DC, Citrus natsudaidai Hayata, Citrus unshiu (Yu. Tanaka ex Swingle) Marcow, and Citrus reticulata Blanco are members of the Rutaceae plant family. Rutaceae members are considered good sources of essential oil. Some family members are Amyris (Amyris balsamifera), Bergamot (Citrus bergamia), Boronia (Boronia megastigma), Cape May (Coleonema album), Clementine (Citrus clementina), Grapefruit (Citrus paradisi), Lemon (Citrus limonum), Lime (Citrus aurantifolia), Mandarin (Citrus reticulata), Neroli (Citrus aurantium var.amara), Orange Blood (Citrus sinensis), Orange Sweet (Citrus sinensis), Petitgrain (Citrus aurantium var. amara), Rue (Ruta graveolens), Tangerine (Citrus reticulata), Tosa Konatsu (Citrus tamurana), Xanthoxylum (Zanthoxylum armatum), Yuzu (Citrus junos), and Zanthoxylum (Zanthoxylum odorum), each of which is a source of essential oils.
This study was undertaken to examine the cytotoxic dosage of essential oils of several Korean native plants, with that dosage based on the IC50 values obtained in human lung (A549) and human skin (Detroit- 551) cells of oils extracted from Cinnamomum loureirii, Juniperus chinensis L., Aster glehnii F. Schmidt, Artemisia capillaris Thunb, Juniperus chinensis L., Juniperus chinensis L. var. sargentii Henry, Citrus natsudaidai Hayata, Citrus unshiu (Yu. Tanaka ex Swingle) Marcow, and Citrus reticulata Blanco. In addition, the cytotoxic mechanisms of the examined essential oils were determined by examining changes in the gene and protein expressions of four cell-cycle markers (Cyclin A, Cyclin B, Cyclin D, and Cyclin E) and one cell-death marker (caspase-3).
2. MATERIALS and METHODS
Plant essential oils are extracted by hydrodistillation with 1 kg of plant leafs and 10L of distilled water on heating mantle (Catalog number: MS-DM608, MTOPS®, Seoul, Republic of Korea) at 102℃. Volatile steam are condensed in dean stark trap. Acquired essential oils are dehydration with sodium sulfite anhydrous, and then stored at 4℃.
A549 (KCLB No: 10185) and Detroit 551 cells (KCLB No: 10110) from Korean cell line bank (Seoul, Republic of Korea) were seeded at a density of 0.5 × 103 cells per well in 6-well plate and the medium used for cell culture was DMEM high glucose media (Catalog number: L0102, Biowest, Nuaillé, France) supplemented with penicillin-streptomycin solution (Catalog number: L0018 Biowest), Plasmocin™ prophylactic (Catalog number: ant-mpp, InvivoGen, San Diego, USA) and fetal bovine serum (Catalog number: 16140071, Thermo Fisher Scientific Korea Ltd., Seoul, Republic of Korea). Cells were incubated in a CO2 atmosphere at 37℃ for 24 h prior to treating with essential oil. The plant essential oils were prepared in four concentrations of 10-8, 10-6, 10-4, and 10-2. After 24 h incubation, the cells are treated with diluted plant essential oil and incubated for 24 h additionally. Subsequently, the plant essential oil and the medium treating the cells were removed and the cells washed with PBS solution (Catalog number: LB 001-02, WELGENE Inc., Gyeongsan, Republic of Korea). EZ-Cytox enhanced cell viability assay reagent (Catalog number: EZ-1000, DoGenBio Co., Ltd., Seoul, Republic of Korea) at the recommended concentration was then placed in each well. One hour later, the absorbance value at 450 nm was measured with an Epoch microplate spectrophotometer (Catalog number: Epoch, BioTek Instruments, Inc., Vermont, USA). Cell viability (%) was determine by using the formula of optical density (OD) of sample treated cells / OD of untreated cells as the control × 100 for each tested concentration. A cell survival curve was calculated from those values, and the IC50 value was determined. After conducting the CCK assay, the same procedure was repeated with plant essential oils that were diluted to concentrations of 10-6, 10-5, 10-4, 10-3, and 10-2.
Cells were seeded in 6-well plates at a density of 0.3 × 10-4 cells per well and incubated at 37℃ in 5% CO2 atmosphere. Plant essential oils in DMEM high glucose media were prepared in three concentrations: IC50 from the CCK assay, 10 times more than the IC50, and 10 times less than the IC50. When cell confluency was 70%, the cells were treated with the appropriate concentration of plant essential oil. Harvesting of cells and RNA extraction were accomplished by using TRI ReagentTM Solution (Catalog number: AM9738, Thermo Fisher Scientific Korea Ltd.) at 24 h after plant essential oil treatment. The purity and concentration of the extracted RNA were measured by using an Epoch microplate spectrophotometer.
The extracted RNA was synthesized by reverse transcription. The concentration of RNA was titrated to 1µg/µL and DEPC-treated water was added to adjust the total volume to 10.5µL. The mixture contained Moloney murine leukemia virus (MMLV) reverse transcriptase (Catalog number: 28025013, Invitrogen, Massachusetts, USA), Ribonuclease inhibitor (Invitrogen), random primers (Catalog number: 3801, TaKaRa, Kyoto, Japan), 100 mM DTT (Invitrogen), dNTP and 5× first strand buffer (Catalog number: 28025013, Invitrogen). The reaction mixture was incubated at 37℃ for 60 min. After incubation, the cells were incubated further at 95℃ for 5 min for inactivation of the enzyme. After cooling for 5 min on ice, the cDNA was stored at -20℃ prior to use.
For real-time polymerase chain reaction (RT-PCR), a mastermix of the following reaction components was prepared: cDNA sample (2 µL), 0.25 µL 50× ROX dye, 6.25 µL 2× prime Q-mastermix SYBR (Catalog number: Q-9210, GeNetBio, Daejeon, Republic of Korea), 0.5 µL forward primer, and 0.5 µL reverse primer. RT-PCR was performed using 7300 RT-PCR system (Applied Biosystems, Foster City, CA, USA) and the procedure sequence was as follows: cDNA denatured at 95℃ for 30 sec and annealed at 58℃ for 30 sec, elongation is performed at 72℃ for 30 sec, and the whole cycle is repeated 40 times. The degree of gene expression was determined by using RQ software (Applied Biosystems).
The primer sequences used are as follows: 5'-CATACTCCACAGCACCTGGTTA-3' (forward) and 5'-CTGTTGCCACCTTTCGGTTA-3' (reverse) for CASP3; 5'-TACTTTCTGCATCAGCAGCC-3' (forward) and 5'-TGATTCAGGCCAGCTTTGTC-3' (reverse) for Cyclin A; 5'-CTTTGCACTTCCTTCGGAGA-3' (forward) and GTAGAGTTGGTGTCCATTCACC-3' (reverse) for Cyclin B; 5'-CCTCGGTGTCCTACTTCA-3' (forward) and 5'-CTCCTCGCACTTCTGTTC-3' (reverse) for Cyclin D; and 5'-GGTTTCAGGGRATCAGTGGT-3' (forward) and 5'-TTTCTTTGCTCGGGGCTTTG-3' (reverse) for Cyclin E. The primer sequence for Rn18s was used as the primer for the control group: 5'-CTCAACAC- GGGAAACCTCAC-3' (forward) and 5'-CGCTCCA- CCAACTAAGAACG-3' (reverse). All primers were obtained from Macrogen (Korea).
Proteins were extracted using Pro-prep (iNtRON Biotechnology, Seongnam, Republic of Korea) according to the manufacturer's instructions. Proteins (30 µg per lane) were separated on a 10% SDS-PAGE gel and transferred to a polyvinylidene fluoride transfer membrane (catalog number: NEF1000, PerkinElmer Inc., Seoul, Republic of Korea) in a TransBlot Cell (catalog number: TE-22; Hoefer Co. Massachusetts, USA) according to the manufacturer's instructions. The resulting blot was blocked with TBS-T containing 5% skimmed milk for 60 min, then incubated with primary antibody: Caspase-3 (mouse monoclonal, 1:500, Catalog number: 9665, Cell Signaling Technology, Massachusetts, USA), Cyclin A2 (mouse monoclonal, 1:500, Catalog number: 4656, Cell Signaling Technology), Cyclin B1 (rabbit polyclonal, 1:500, Catalog number: 4138, Cell Signaling Technology), Cyclin D1 (rabbit monoclonal, 1:500, Catalog number: 2978, Cell Signaling Technology), Cyclin E1 (mouse monoclonal, 1:500, Catalog number: 4129, Cell Signaling Technology), or β-actin (rabbit monoclonal, 1:1,000, Catalog number: 4970, Cell Signaling Technology). After washing in buffer, the membranes were incubated with the appropriate horseradish peroxidase-conjugated secondary antibodies (anti-goat, 1:2,000, Catalog number: sc-2354 or anti-mouse, 1:4,000, Catalog number: sc-2005, Santa Cruz, Texas, USA) for 1 h at room temperature. Next, the blots were developed by incubation in ECL chemiluminescence reagent (Catalog number: sc-2048, Santa Cruz) and subsequently exposed to the GeneGnome Bioimaging System (Catalog number: 77534-3, Syngene, Maryland, USA) for development, after which images were captured by using the GENESys software (Syngene). Signal specificity was confirmed by blotting in the absence of primary antibody, and bands were normalized to β-actin immunoreactive bands visualized on the same membrane after stripping. The density of each band was measured using the NIH ImageJ software.
All data were analyzed by applying nonparametric one-way analysis of variance followed by Tukey’s test for multiple comparisons. All experiments consisted of three separate trials. Statistical analysis was performed by using Prism Graph Pad (v.8.0; GraphPad Software, San Diego, USA).
3. RESULTS and DISCUSSION
The CCK assay was used to obtain IC50 values of each essential oil. To determine that concentration, cells were treated with four concentrations of each essential oil, followed by a further subdivision of the doses using the CCK assay. The IC50 values for the ten plant essential oils tested are presented in Fig. 1 and 2. In the Detroit-551 and A549 cell lines, the degree of cytotoxicity of each essential oil was compared with its IC50 value. The essential oil IC50 values obtained from Detroit-551 cell assays were Artemisia capillaris Thunb. (5.4510-3), Aster glehnii F. Schmidt (2.9410-3), Zanthoxylum piperitum (L.) DC (1.6410-2), Zanthoxylum schinifolium Siebold & Zucc. (1.5610-2), Juniperus chinensis L. (4.5310-3), Juniperus chinensis L. var. sargentii Henry (5.5910-3), Cinnamomum loureirii Nees (1.0910-4), Citrus reticulata Blanco (2.7710-3), Citrus natsudaidai Hayata (5.8010-3), and Citrus unshiu (Yu. Tanaka ex Swingle) Marcow (3.1610-3). Similarly, the essential oil IC50 values obtained from A549 cell assays were Artemisia capillaris Thunb. (1.9110-3), Aster glehnii F. Schmidt (2.7710-3), Zanthoxylum piperitum (L.) DC (1.5910-2), Zanthoxylum schinifolium Siebold & Zucc. (1.3010-2), Juniperus chinensis L. (2.7910-3), Juniperus chinensis L. var. sargentii Henry (4.1510-3), Cinnamomum loureirii Nees (7.4610-4), Citrus reticulata Blanco (1.0210-3), Citrus natsudaidai Hayata (6.5910-3), and Citrus unshiu (Yu. Tanaka ex Swingle) Marcow (2.3410-3).
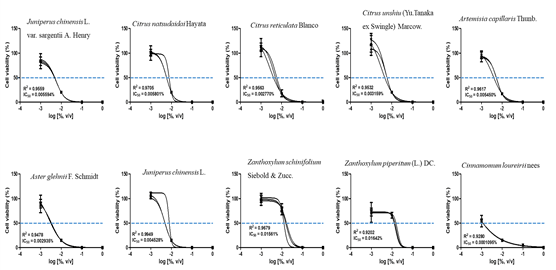
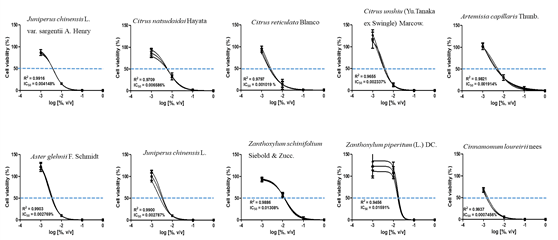
The expression levels of CASP3, a marker gene related to cellular apoptosis, were determined by performing quantitative RT-PCR of cells after essential oil treatment. The expression levels of CASP3 in the A549 and Detroit-551 cells after treatment with each of the ten plant essential oils are shown in Fig. 3. The expression of CASP3 in Detroit-551 cells increased at concentrations near the IC50 values of Citrus reticulata Blanco, Citrus unshiu (Yu. Tanaka ex Swingle) Marcow, Aster glehnii F. Schmidt, Zanthoxylum schinifolium Siebold & Zucc, Cinnamomum loureirii Nees. However, none of the ten essential oils increased CASP3 mRNA expression in A549 cells.
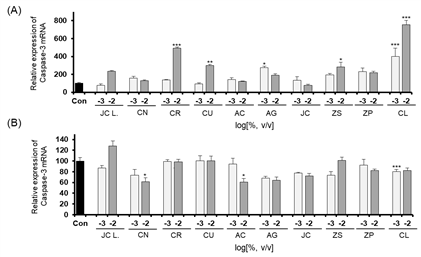
The expression levels of Cyclin A, Cyclin B, Cyclin D, and Cyclin E when Detroit-551 cells were treated with 10-2 and 10-3 % (v/v) concentrations of the ten essential oils are shown in Fig. 4. The cell-cycle control gene, Cyclin A, showed an increased expression following Cinnamomum loureirii Nees treatment. Cyclin B showed increased expression levels following treatment with essential oils of Citrus reticulata Blanco, Zanthoxylum piperitum (L.) DC, Cinnamomum loureirii Nees. Zanthoxylum schinifolium Siebold & Zucc., and Zanthoxylum piperitum (L.) Only the Zanthoxylum piperitum (L.) DC treatment produced an increased level of Cyclin D expression, and Cinnamomum loureirii Nees was the only essential oil that induced an increase in Cyclin E expression.
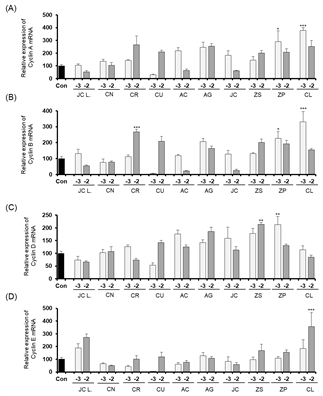
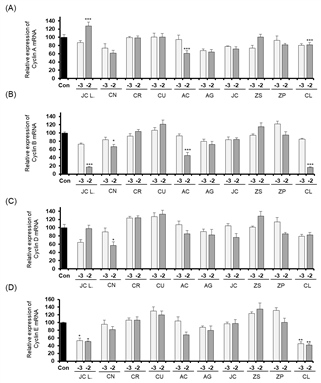
In the A549 cell line, only Juniperus chinensis L. var. sargentii Henry treatment (10-2 % v/v concentration) induced an increase in Cyclin A expression. None of the ten essential oil treatments increased the expressions of cyclins B, D, and E in A549 cells.
At the protein level, expressions of cyclins A and Cyclin B were increased in Detroit-551 cells, results considered to indicate the cells were in mitosis or the S phase of the cell cycle. Cyclin B was increased following Citrus unshiu (Yu. Tanaka ex Swingle) Marcow, Artemisia capillaris Thunb, Aster glehnii F. Schmidt, and Cinnamomum loureirii Nees treatments. Notably, caspase-3 expression was increased in Detroit-551 cells treated with Juniperus chinensis L. var. sargentii Henry, Citrus unshiu (Yu. Tanaka ex Swingle) Marcow, Artemisia capillaris Thunb, Juniperus chinensis L, and Cinnamomum loureirii Nees. In the treated A549 cells, Cyclin A expression was increased with Citrus reticulata Blanco, Citrus unshiu (Yu. Tanaka ex Swingle) Marcow, Zanthoxylum schinifolium Siebold & Zucc, Zanthoxylum piperitum (L.) DC, and Cinnamomum loureirii Nees treatments. In addition, caspase-3 expression increased with Juniperus chinensis L. var. sargentii Henry, Citrus natsudaidai Hayata, Citrus reticulata Blanco, Citrus unshiu (Yu. Tanaka ex Swingle) Marcow, Artemisia capillaris Thunb, and Juniperus chinensis L. treatments.
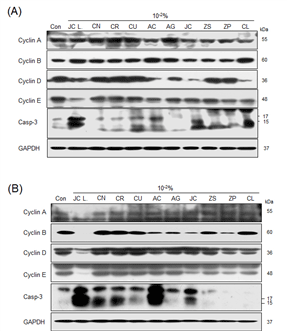
Essential oils have been used in traditional medicines, and systematic, scientific examination of the biological activities of essential oil from plants has been conducted for several decades (Borges et al., 2005; Mohammadhosseini et al., 2017). Traditional herbal medicines, including essential oils, have been used for treating infections and modulating various physiological functions (Horvath et al., 2015). Essential oils also have great potential as anti-inflammatory, anti-bacterial, and anti-cancer therapeutic agents (Bravo et al., 1980; Pagano et al., 1992; Elledge, 1996). However, dosages and the methods for oil extraction are inconsistent, which can result in cytotoxic effects when using essential oils. In addition, reports describing the mechanisms of essential oil cytotoxicity are lacking. Indeed, the complex chemical composition of plant essential oil extracts makes it difficult to evaluate a mechanism of action underlying the biological effects such as cell toxicity, cytostatic effects, and side effects (Ahn et al., 2020).
The cytotoxic mechanisms associated with plant essential oils are typically divided into two types: apoptosis and necrosis; both of which are cytotoxic mechanisms that can kill cells or inhibit cell growth by delaying the cell cycle and inhibiting cell proliferation.
The cell cycle is divided into the S phase, the time when DNA is doubled, the M phase, when chromosome distribution and cell division occurs, and the G1 and G2 phases, which occur between these S and M phases; the overall cycle is a periodic sequence of phases G1, S, G2, and M (Gerdes et al., 1984). Each phase of the cell cycle and its progression to the next phase are regulated by a complex of cyclins and cyclin-dependent kinases (CDK) (Peter et al., 1994). In the G1 phase, Cyclin D/CDK4/6 and Cyclin E/CDK2 act in sequence (Peter et al., 1994). Cell-cycle regulation maintains genome stability by checking for genome damage and ensuring the cellular DNA is replicated during one cell cycle before division into two daughter cells (Bartek et al., 2004). If the cell cycle and checkpoint system are not functioning normally, cells may die or cause chromosome instability, resulting in fatal diseases such as cancer (Gerlach et al., 2020). Cells that do not undergo cell division exhibit apoptosis or programmed cell death. When cell death occurs, a signal is transmitted to neighboring cells (Kawamoto et al., 2016). Malfunctioning cells that undergo apoptosis or death are beneficial to the survival of an organism. In addition, recent reports have defined many roles for apoptosis in normal lung function and in pathophysiology unrelated to lung neoplasia (Schittny et al., 1998). Detroit-551 cells are representative of epithelial cells, such as skin cells, whereas A549 cells are adenocarcinomic human alveolar basal epithelial cells, have been used in type II cell study, and are sensitive to lung damage. Using these two cell types, we examined the cytostatic and cytotoxic effects of essential oils of ten Korean native plants.
In this study, by determining IC50 values and the expression levels of cellular apoptosis marker caspase-3 and cell-cycle markers cyclins A, B, D, and E, we examined the cell number reduction effects in ten essential oils at concentrations near the IC50 concentration. The apoptosis marker CASP3 displays an increase in gene expression upon apoptosis; thus, we evaluated cytotoxic effects based on protein expression levels. At the protein level, caspase-3 expressions were increased due to Juniperus chinensis L. var. sargentii Henry, Citrus unshiu Marcow, Artemisia capillaris Thunb, Juniperus chinensis L, Cinnamomum loureirii Nees in Detroit-551. Otherwise, caspase-3 expressions were increased by Juniperus chinensis L. var. sargentii Henry, Citrus natsudaidai Hayata, Citrus reticulata Blanco, Citrus unshiu Marcow, Artemisia capillaris Thunb, Juniperus chinensis L. Those results indicates that the decrease of the cell proliferation near IC50 value was from increased level of caspase-3 induced cell death by those essential oils treatment.
A relatively high dose of essential oil (10-2) induced Cyclin A increases in Detroit-551 cells, which is indicative of mitosis or the S phase arrest in the cell cycle. M phase arrest may induced by Citrus unshiu (Yu. Tanaka ex Swingle) Marcow, Artemisia capillaris Thunb, Aster glehnii F. Schmidt, and Cinnamomum loureirii Nees treatment in order to increased Cyclin B. Similarly, in A549 cells, Cyclin A was increased with Citrus reticulata Blanco, Citrus unshiu (Yu. Tanaka ex Swingle) Marcow, Zanthoxylum schinifolium Siebold & Zucc, Zanthoxylum piperitum (L.) DC, and Cinnamomum loureirii Nees treatments.
In shorts, essential oil from Juniperus chinensis L. var. sargentii Henry, Juniperus chinensis L. induce cell death by apoptosis in order to increase expression of caspase-3. Citrus unshiu, Artemisia capillaris Thunb essential oils induce cell death by cytostatic effects in order to cell cycle arrest and inducing apoptosis. Citrus natsudaidai Hayata, Citrus reticulata Blanco, Cinnamomum loureirii Nees essential oils can induce both cytostatic and apoptotic effect depends on cell type. Other essential oil from Aster glehnii F. Schmidt, Zanthoxylum schinifolium Siebold & Zucc., Zanthoxylum piperitum (L.) DC induce only cytostatic effect in order to cell cycle arrest. This result is need to be considered before development and application of these essential oils.
4. CONCLUSION
Based on the results, essential oils from various plants can produce cytotoxic and cytostatic effects. Such effects should be evaluated further to predict potential side effects of the clinical usage of essential oils. The pharmacological properties of essential oils depend on their chemical contents and the concentrations used. Careful selection of a treatment concentration is important when using plant essential oils for medicinal or cosmetic purposes.