1. INTRODUCTION
As fishing technology has been advanced, it has become large in scale and mass production is possible. However, high density fish farming in a limited place causes problems such as environmental pollution because it is difficult to manage the surrounding water quality. This increases more frequent infection with fish pathogens and causes massive economic loss because the disease quickly spreads in small places (Oh et al., 2006; Yan and Kim, 2013).
Although there are differences in bacterial diseases according to fish species, recently, damage such as edwardsiellosis mainly caused by Edwardsiella tarda, streptococcosis caused by Streptococcus parauberis and Lactococcus garvieae, and vibriosis caused by Photobacterium damselae has been reported (Jee et al., 2014; Kim et al., 2015). These are representative infectious pathogenic organisms that cause bacterial diseases of olive flounder, and olive flounder infected with bacteria shows symptoms such as bleeding, abdominal distension, and enlarged kidneys, eventually leading to death (Han et al., 2006; Cho et al., 2007; Moon et al., 2009; Nho et al., 2009). In addition, some bacteria including E. tarda, are known as bacterial zoonoses, which can infect humans as well as fish and cause disease (Erfanmanesh et al., 2012; Hundenborn et al., 2013; Hirai et al., 2015; Choksi and Dadani, 2017). Accordingly, antibiotics were administered to feed or water to lower the death rate of fish and increase fish production by treating or preventing diseases caused by fish pathogens (Markestad and Grave, 1997; Cabello, 2006). However, continued use of antibiotics and indiscriminate misuse and abuse are likely responsible for not only leading to emergence of resistant strains, but also disturbing environmental ecosystems (Rhodes et al., 2000; Cabello, 2006). Therefore, development of alternative antibiotic materials of natural origin has received attention to minimize side effects caused by the use of such antibiotics and effectively control fish pathogens (Grenni et al., 2017; Rossiter et al., 2017).
Essential oil, a natural resource, is one of the secondary metabolites isolated from scented plants, and it is a volatile mixture in the form of oil. It is known that essential oils have various biological effects such as antioxidant (Amorati et al., 2013; Salgado-Garciglia et al., 2018; Jeong et al., 2017), antifungal (Nazzaro et al., 2017; D’agostino et al., 2019), insecticidal (Tripathi et al., 2009; Ayvaz et al., 2010), and antibacterial activity (Bakkali et al., 2008; Nazzaro et al., 2013; Chouhan et al., 2017). Studies have also been conducted to analyze the ingredients and to evaluate the toxicity for their safety (Min et al., 2017; Ahn et al., 2018). Pinaceae is a representative plant with a high essential oil content, and it mainly consists of volatile aromatic compounds of terpene, such as monoterpenes and sesquiterpenes containing hydrocarbons and oxygenated derivatives (Koukos et al., 2000; Hong et al., 2004). Essential oils from Pinaceae family are widely used in aromatherapy (Ali et al., 2015), anti- inflammation (Yang et al., 2019), antioxidation (Xie et al., 2015), and antimicrobial activity (Chouhan et al., 2017), etc (Aziz et al., 2018). In particular, pine essential oil, also called phytoncide, has strong antibacterial activity against various gram-negative and gram-positive bacteria (Mohammed et al., 2001; Lee et al., 2014).
Although there have been studies on antibacterial activity against fish pathogens of natural products including essential oils (Bulfon et al., 2013; Park et al., 2016; Cunha et al., 2018), no demonstration has been made on the efficacy of essential oils from Pinaceae family to date. In addition, most studies have compared and evaluated the efficacy of essential oils according to the sample region or extraction method, or presented the main components through analysis. However, since various components are mixed in essential oils, there are cases where it is impossible to explain them with one component, and there have been very few studies on important active components that explain these specific phenomena.
For that reason, in this study, we evaluate antibacterial activity of essential oils from Pinaceae leaves, which are A. holophylla, P. thunbergii, P. parviflora, T. sieboldii, and P. rigitaeda, against fish pathogens and investigate their own active ingredients. Through this, we tried to investigate whether essential oils from Pinaceae leaves can be used as a prevention of fish pathogens or as a medicine for fisheries.
2. MATERIALS and METHODS
All five trees used in the study belong to Pinaceae family. The leaves of A. holophylla, P. thunbergii, and P. rigitaeda were collected from the National Institute of Forest Science in Suwon City, T. sieboldii was collected from the National Institute of Forest Science in Pocheon-eup, and P. parviflora leaves from Ulleungdo. And, all reagents used in the study are from Sigma-aldrich; (-)-bornyl acetate (99%, product number: 45855), α-pinene (97%, product number: 80604), (-)-borneol (99%, product number: 15598), neryl acetate (97%, product number: 46015), and (-)-carveol (98%, product number: 61370).
Essential oils were extracted using hydro-distillation from the leaves of A. holophylla, P. thunbergii, P. parviflora, T. sieboldii, and P. rigitaeda. 5 to 6 L of distilled water per 1 kg of sample was added to a 10 L volume round flask. Extraction was carried out until no more essential oil was extracted with a heating mantle (Model: MS-DM608, Serial number: 201602, Misung Scientific. Co. Ltd., Korea) at 100 ± 2 °C. The extracted essential oil was dehydrated using anhydrous Na2SO4 (98.5%, Samchun, Korea) and filtered using 0.45 μm pore size minisart® syringe filter (Reference number: 16555-K, Sartorius Stedim Biotech GmbH, Germany). The filtered essential oil was transferred to a light-blocked brown bottle, and then filled with nitrogen gas and stored in a 4 °C refrigerator until use.
Edwardsiella tarda FP5060, Photobacterium damselae FP4101, Lactococcus garvieae FP5245, and Streptococcus parauberis FP3287 were received from Marine and Fisheries Life Resources, National Institute of Fisheries Sciences, and they were stored in 25% glycerol stock at -40°C. BHI agar was prepared by adding 15 g/L agar to BHI broth (Bacto™ brain heart infusion, Product number: 237500, BD Biosciences Korea Ltd., Korea). The frozen storage bacteria were inoculated on a BHI agar plate and cultured at 28 °C for 24 hours to ensure a single colony. A single colony was inoculated into 4 mL BHI broth, and then cultured with 250 rpm for overnight.
The bacteria cultured in BHI broth were measured at 600 nm using a Neo-D3117 UV-VIS spectrophotometer (NEOGEN Inc., Korea), and optical density (O.D) was adjusted to 1 (O.D600 = 1). After inoculating 100 μL of the concentration-adjusted bacteria in the center of Mueller hinton agar (Difco, Product number: 225250, BD Biosciences Korea Ltd., Korea) plate, it was spread evenly using a glass spreader. A sterilized paper disc (ADVANTEC, Product number: 49005040, Toyo Roshi Kaisha, Ltd. Japan) was attached to the plate, and each essential oil stock solution was absorbed by 5 μL. After that, the cells were cultured at 28 °C for 2 days, and measure the diameter of a ring formed around the paper disc to evaluate antibacterial activity.
The microdilution method of CLSI M07-A9 was modified and used in order to evaluate the antibacterial activity. A. holophylla, P. thunbergii essential oils and a single compound were dissolved in dimethyl sulfoxide (DMSO, extra pure grade, Product number: 3047-4460, Duksan) and were prepared at a concentration of 5% (v/v) until use. Essential oil samples were diluted to 3.5, 2, 1, and 0.5% concentration. 200 μL of BHI broth was dispensed into each well of a 96-well polystyrene microplate (Flat bottom well, Product number: 30096, SPL Life Sciences Co., Ltd., Pocheon, Korea). The prepared sample was treated with 2 μL of each well. Then, each well was inoculated so that the concentration of pre-cultured bacteria was O.D600= 0.05, and cultured at 28 °C for 24 hours. After incubation for 24 hours, the absorbance was measured at 600 nm using an Epoch microplate spectrophotometer (BioTek Instruments, Inc., US) to evaluate the antibacterial activity of essential oils. The control group was treated with 1% DMSO in which the sample was dissolved, and the positive control group was tetracycline (HPLC grade, Product number: 87128, Sigma-Aldrich, Korea), an antibiotic.
The components of essential oils extracted from A. holophylla and P. thunbergii leaves were analyzed using GC-MS analysis. For GC-MS analysis, TRACE™ 1310 Gas Chromatograph consisting of TriPlus™ 100 LS Liquid Auto-sampler (Catalog number: IQLAAAGAAHFACMMBES), ISQ™ Series Single Quadrupole GC-MS System (Catalog number: IQLAAAGAAJFALOMAYE) was used, and all are Thermo Fisher Scientific products (Thermo Fisher Scientific Solutions LLC, Korea). For the column, VF-5MS (Silica, length 60 m x diameter 0.25 mm x thickness 0.25 μm, Agilent Technologies, Inc., US) was used. Helium was supplied as a mobile phase gas at a rate of 1 mL/min. The essential oil sample was dissolved 4 μL in 1 mL of dichloromethane and injected 1 μL. Injection temperature was maintained at 250 °C, and a split ratio mode of 1:20 was used. Oven temperature was initially maintained at 50 °C for 5 minutes, then increased to 65 °C at 10 °C/min and maintained for 30 minutes. Thereafter, the temperature was raised to 210 °C at 5 °C/min and maintained for 10 minutes. Lastly, the temperature was increased up to 325 °C at 20 °C/min and maintained for 10 minutes. The temperature of the flame ionization detector (FID) was set to 300 °C, the air flow was set to 350 mL/min, the hydrogen flow to 35 mL/min, and the make-up gas (helium) to 40 mL/min. MS data were collected in the range of 35 - 550 amu at 0.2 sec/scan in EI ionization mode.
Among the collected peak data, those with high match quality were first selected, and Kovats retention index (KI) was calculated using n-alkanes (C8 - C20, Product number: 04071, Sigma-Aldrich, Korea). The calculated KI value was compared with the National Institute of Standards and Technology (NIST, US) Chemistry WebBook (Standard Reference Database Number 69), and we finally selected compounds with a difference of less than 100.
All experimental results are presented as mean and standard deviation. One way analysis of variance was used to distinguish significant differences using IBM® SPSS software (Ver. 25.0; SPSS Inc., Chicago, Illinois, USA). Significance levels were set at 95% confidence intervals for Tukey test or paired-t-test.
3. RESULTS and DISCUSSION
The yields of each essential oil extracted from A. holophylla, P. thunbergii, P. parviflora, T. sieboldii, and P. rigitaeda leaves were found to be 2.99%, 0.93%, 0.08%, 0.15%, 0.72%, and the antibacterial effect was evaluated with the paper disc diffusion method. The presence of antibacterial activity of essential oils was confirmed by measuring the size of the ring formed by exposing 4 types of fish pathogens to a paper disc containing essential oils for 2 days (Table 1). A clear zone arising around the paper disc is formed because bacteria cannot grow in the area, so the size of the growth inhibiting ring indicates an index of antibacterial activity (Djabou et al., 2013).
For E. tarda, A. holophylla and P. thunbergii essential oils showed strong antibacterial activity with 21.5 mm and 21.0 mm, respectively, followed by T. sieboldii (16 mm) and P. rigitaeda (13 mm) in order. Also, for P. damselae, A. holophylla and P. thunbergii essential oils formed a wide growth-inhibiting ring of size 23.5 mm and 20.5 mm, followed by T. sieboldii (13.25 mm), P. rigitaeda (11.5 mm), and P. parviflora (8 mm) in order. The antibacterial effect of tested essential oils against L. garvieae was lower than that of E. tarda and P. damselae, and among 5 essential oils, A. holophylla essential oil was 12.75 mm, which showed higher antibacterial activity than other essential oils. For S. parauberis, P. thunbergii essential oil formed the widest ring of 14.0 mm, while the remaining essential oil formed a growth inhibitory ring of similar size at the level of about 10 mm. On the other hand, P. parviflora essential oil did not form rings against E. tarda and S. parauberis. From these results, we noticed that the essential oils of A. holophylla and P. thunbergii leaves had a strong antibacterial effect against fish pathogens, and were particularly effective against E. tarda and P. damselae.
As a result of the paper disc assay, E. tarda and P. damselae, the gram-negative bacteria, showed high sensitivity to essential oils of A. holophylla and P. thunbergii leaves. In contrast, the gram-positive bacteria L. garvieae and S. parauberis were relatively less susceptible to both essential oils (Table 1). Therefore, we investigated the growth change according to the concentration of essential oils of A. holophylla and P. thunbergii leaves against 4 types of fish pathogens (Fig. 1). For E. tarda, A. holophylla leaves essential oil inhibited growth of about 5% at a concentration of 0.01%, and its effect increased rapidly at a concentration of 0.02% to inhibit 100%. P. thunbergii leaves essential oil showed a growth inhibition effect of about 10% and 25% at concentrations of 0.01% and 0.02%, respectively, and 100% effect at concentration of 0.035% (Fig. 1A). The growth of P. damselae was rapidly inhibited in the essential oil of A. holophylla and P. thunbergii leaves at 0.02% concentration, and the inhibitory effects were 74% and 83%, respectively. In addition, 0.035% concentration of A. holophylla leaves essential oil showed inhibitory effect on the growth of about 93% and P. thunbergii leaves essential oil did about 81%. The essential oils of A. holophylla and P. thunbergii leaves had little effect on the growth of L. garvieae (Fig. 1C). S. parauberis growth was also not affected by A. holophylla leaves essential oil, but growth was inhibited by about 18% by 0.035% concentration of P. thunbergii leaves essential oil (Fig. 1D). Such antibacterial research on fish pathogens of A. holophylla and P. thunbergii leaves essential oil has not been reported so far, and it is significant in that it suggested the possibility of them as a material for preventing infectious diseases against fish pathogenic bacteria.
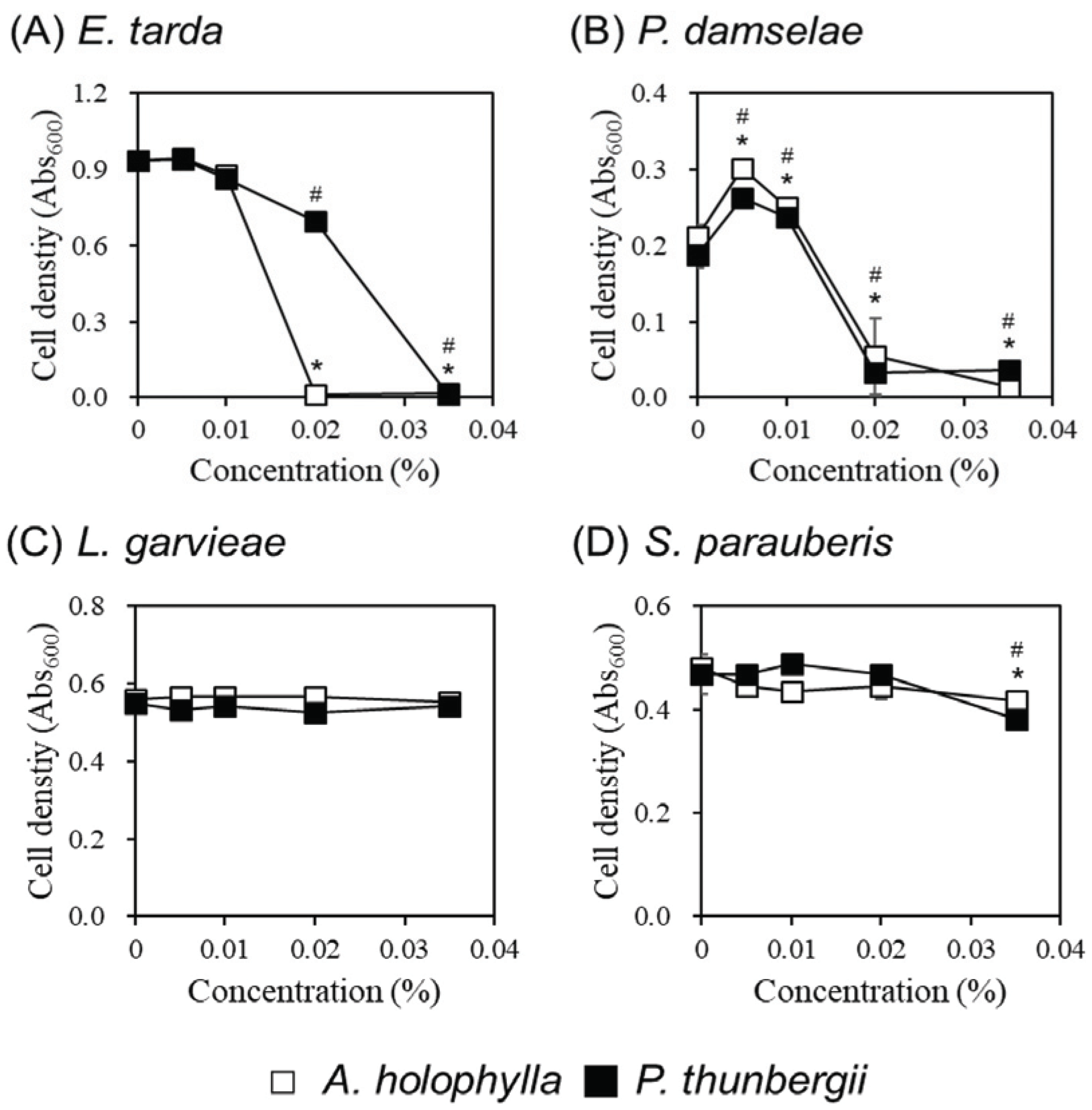
In general, the antimicrobial activity of essential oils is known to be more effective against gram-positive bacteria than gram-negative bacteria (Trombetta et al., 2005; Nazzaro et al., 2013). In gram-positive bacteria, about 90 - 95% of the cell wall is composed of peptidoglycan, and teichoic acid and lipoteichoic acid are present on the cell wall surface. Therefore, the cell wall of gram-positive bacteria allows hydrophobic substances such as essential oil to pass relatively easily, and can act on the cell wall or the cytoplasm inside the cell (Tiwari et al., 2009). On the other hand, the cell wall of gram-negative bacteria has a thinner layer of peptidoglycan than gram-positive bacteria, and there is one more outer membrane on the outermost side. It is known that the lipopolysaccharide present in the outer membrane gives hydrophilic properties to the surface of the cell wall, and thus exhibits resistance to hydrophobic substances such as essential oils (Vaara, 1992; Nikaido, 1994; Mann et al., 2000). Previous studies have also reported that essential oils extracted from herbaceous plants are more effective in inhibiting the growth of gram-positive bacteria than in gram-negative bacteria. (Aumeeruddy Elalfi et al., 2015; Martucci et al., 2015; Bouazama et al., 2017), Also, essential oils of woody species have been reported to show MIC levels at lower concentrations for gram-positive bacteria (Aumeeruddy Elalfi et al., 2015). There was one study in which time-kill analysis was performed using essential oils extracted from Citrus medica. As a result, Escherichia coli was inhibited to a maximum growth 4 hours after exposure to essential oils, but Staphylococcus aureus was found to be 2 hours earlier than this (Li et al., 2019).
Interestingly, however, the essential oils from A. holophylla, P. thunbergii, P. rigitaeda, and T. sieboldii leaves tested in this study were more effective and showed high antibacterial activity against gram-negative bacteria (E. tarda and P. damselae) than gram-positive bacteria (L. garvieae and S. parauberis) (Table 1). Particularly, MIC concentrations of E. tarda and P. damselae were confirmed by the essential oils of A. holophylla and P. thunbergii leaves, which showed the strongest growth inhibitory effect, but the effect was insufficient at all concentrations for gram-positive bacteria (Fig. 1). These results are in contrast to the previously reported research results, and we determined that it was necessary to analyze composition of the essential oils of A. holophylla and P. thunbergii leaves.
We analyzed compound composition of the essential oil extracted from the leaves of the five species belonging to Pinaceae family using GC-MS analysis (Table 2). The major components of the essential oil of P. parviflora leaves were α-pinene (36.58%), β-pinene (23.49%), and D-limonene (18.99%). And T. sieboldii leaves had (-)-bornyl acetate (47.06%), α-pinene (12.03%), and camphene (8.42%) as major components. In addition, the ratio of β-pinene (18.08%) and α-pinene (8.19%) was high in P. rigitaeda.
![]() |
Most of the components of the essential oils of A. holophylla and P. thunbergii leaves, which have a strong antibacterial activity against gram-negative bacteria, were analyzed as terpene-based compounds, including monoterpene and sesquiterpene. The main components of A. holophylla leaves essential oil were 22 monoterpene (79.82%) and 7 sesquiterpene (7.21%), and the main constituents of P. thunbergii leaves essential oil were 26 monoterpene (80.94%) and 9 sesquiterpene (14.00%). The main components of A. holophylla leaves essential oil were (-)-bornyl acetate (29.45%), D-limonene (20.47%), camphene (11.73%), and α-pinene (6.08%). P. thunbergii leaves essential oil had the highest ratio of α-pinene at 59.81%, followed by caryophyllene oxide (5.44%), D-limonene (4.34%), and β-myrcene (4.24%). A total of 17 compounds were found in both essential oils of A. holophylla and P. thunbergii leaves, and 12 were monoterpene and 5 were sesquiterpene. From these results, we identified that (-)-bornyl acetate and α-pinene account for the largest proportion of the two essential oils extracted by hydro-distillation in this study. Other studies suggest bicyclo [2.2.1] heptan- 2-ol (28.05%), δ3-carene (13.85%), and α-pinene (11.68%) as the main components of A. holophylla leaves essential oils (Lee and Hong, 2009), or does 3-carene (25.53%), α-pinene (17.55%), and bornyl acetate (16.22%) (Kim et al., 2016). P. thunbergii essential oil also reported 2H-benzocyclohepten-2-one (34.33%), α-humulene (19.59%), limonene (5.92%) and caryophyllene (5.32%) as the main components. (Kim et al., 2013). For extracts including essential oils, there are differences in components due to various extraction conditions such as the sample site, harvest time, extraction method, and temperature and time during extraction (Tongnuanchan and Benjakul, 2014; Lingan, 2018). Therefore, these factors make a difference in the compounds separated from plants, and we assume that the final composition ratio of essential oils is likely to be affected by them. Accordingly, for future research, it requires standardization of an appropriate extraction method in order for essential oils to be used as a functional material, and needs to present each of the indicators that serves as a standard for quality through an analysis of essential oils representing physiological activity.
Various compounds exist in plant extracts including essential oils, and since they act organically and exhibit physiological activity, it is difficult to clearly present the mechanism (Sutili et al., 2016). In addition, there are cases where a compound known as a standard component does not match the efficacy of the extract, but rather the phenomenon may be explained by a trace amount of the compound (Chouhan et al., 2017; Ham and Kim, 2019).
In this study, we evaluated the activity of the terpene- based single compound constituting the essential oil, and sought to identify an active ingredient exhibiting similar physiological activity to the essential oils. We carried out antibacterial screening of various terpene- based single compounds (0.05% concentration) including the most existing (-)-bornyl acetate and α -pinene based on the results of GC-MS analysis of the essential oils of A. holophylla and P. thunbergii leaves (Data not shown). As a result, three monoterpenebased active compounds with neryl acetate, (-)-borneol, and (-)-carveol, which selectively inhibit the growth of E. tarda and P. damselae, are obtained in the same effect as essential oils of A. holophylla and P. thunbergii leaves (Fig. 2). E. tarda was inhibited by 68%, 75%, and 69% of growth by three compounds and 77% by tetracycline (0.0005% concentration), which was a positive control (Fig. 2A). P. damselae also inhibited 55%, 70%, and 71% of growth by three single compounds, but only 17% by tetracycline (Fig. 2B). On the other hand, the effects of three terpene- based compounds had insignificant impact on growth of the gram-positive bacteria L. garvieae and S. parauberis. L. garvieae showed a 10% of growth reduction only by (-)-borneol and 42% of inhibition by tetracycline (Fig. 2C). There was no compound that inhibited the growth of S. parauberis, and only tetracycline, which was a positive control, showed 81% of an inhibitory effect (Fig. 2D).
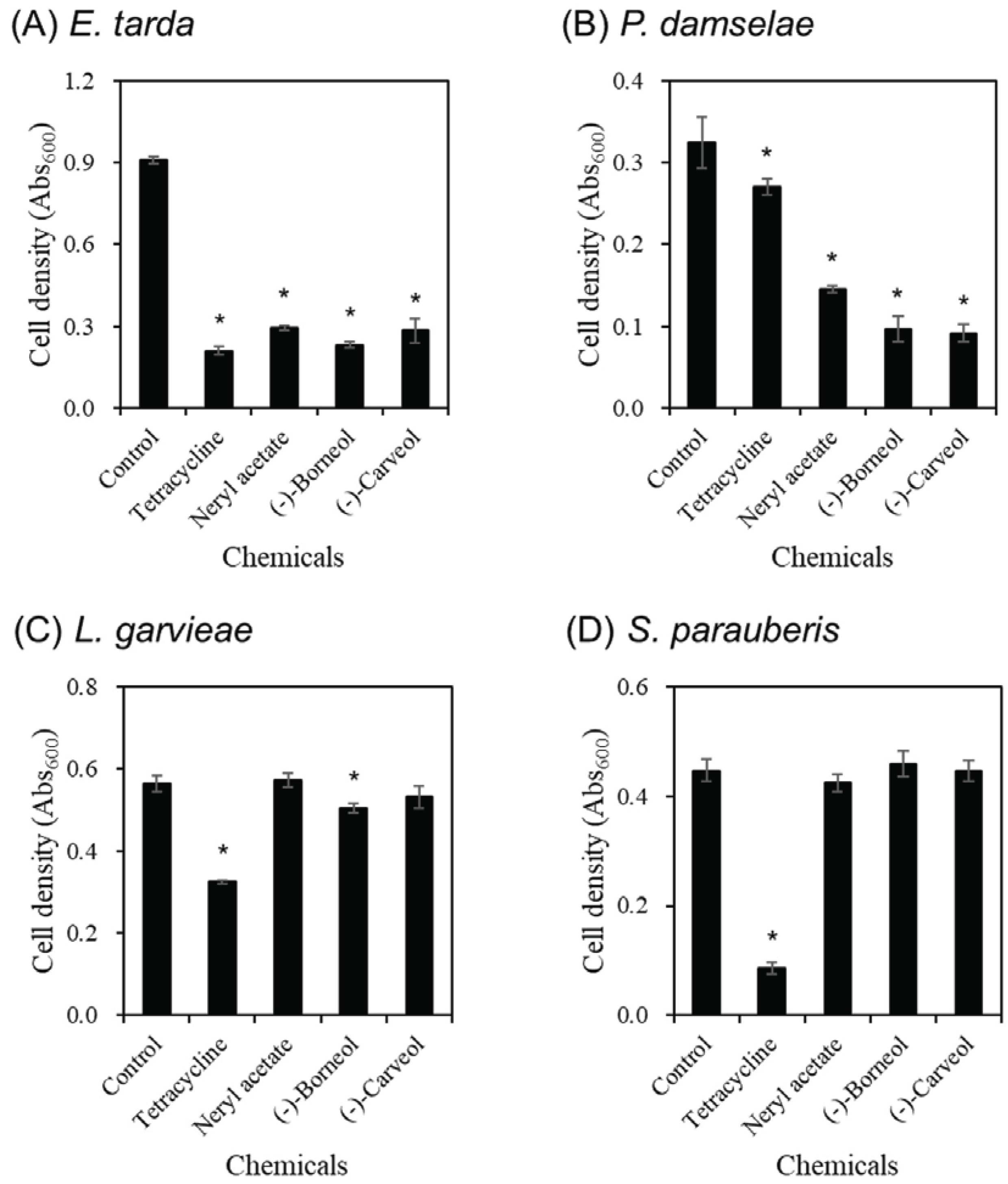
The results of the efficacy evaluation of neryl acetate, (-)-borneol, and (-)-carveol have significance in providing fundamental data that explains specific effects of essential oils of A. holophylla and P. thunbergii leaves, which shows strong and selective antibacterial activity against gram-negative fish pathogens.
Neryl acetate and (-)-carveol were found only in A. holophylla leaves essential oils, and (-)-borneol was detected in both A. holophylla and P. thunbergii leaves essential oils (Table 2). These results may partially explain the phenomenon that A. holophylla leaves essential oil has a superior growth inhibition effect against E. tarda than P. thunbergii leaves essential oil. On the other hand, neryl acetate and (-)-borneol were also detected in essential oils of P. rigitaeda leaves, but did not have strong antibacterial activity against fish pathogens. Some studies have combined the components present in the extract, confirming that there is a synergistic effect between single compounds. (Ham and Kim, 2019; Kim et al., 2016). From these studies, it also showed a synergistic effect of antibacterial activity between neryl acetate, (-)-carveol, (-)-borneol, and other components in the essential oils of A. holophylla and P. thunbergii leaves, and it is presumed to have high antibacterial activity when compared to P. rigitaeda.
Prior studies on (-)-borneol and (-)-carveol have been shown to have antibacterial (Knobloch et al., 1989; Hammerschmidt et al., 1993; Tabanca et al., 2001; Cha, 2007; Jung, 2009; Lopez-Romero et al., 2015; Guimaraes et al., 2019) as well as antifungal effects (Tabanca et al., 2001; Hussain et al., 2010), and neryl acetate has also been reported to inhibit the growth of some pathogenic microorganisms found in industry (Kotan et al., 2007). However, studies on fish pathogens with these compounds have not been conducted, and such previous studies have not provided results on specific antibacterial activity by species classification. Given these results, this study suggests the possibility of selectively controlling gram-negative fish pathogens by using neryl acetate, (-)-borneol and (-)-carveol, which are classified as oxygenated monoterpene.
Generally, mechanisms of essential oils for microorganisms are known to destroy cell membranes (Li et al., 2014; Raeisi et al., 2015), loss of membrane integrity (Diao et al., 2014; Yang et al., 2015), and increase in permeability of cell membranes (Lambert et al., 2001; Hyldgaard et al., 2012) and the cytoplasm is transformed by essential oils introduced into the cell and eventually it leads to cell death (Nazzaro et al., 2013). In addition, essential oil is known to affect expression of pathogenic factors (biofilm, spore formation and mating) by acting on a quorum sensing system, which plays an important role in the interaction between bacteria (Bouyahya et al., 2017). In the case of gram-negative bacteria with high resistance to hydrophobic molecules, it has been reported that some hydrophobic compounds such as essential oils can slowly pass through the porin protein present in the cell wall (Plesiat and Nikaido, 1992; Bock and Sawers, 1996). Based on these previous studies, further studies are needed about additional mechanisms for the gram-negative fish pathogens of essential oil and three oxygenated terpenes obtained in this study. In this study, we found a material that is selectively applied only to a specific species among various fish pathogens that are problems in aquaculture. Also, we anticipate that this is a new eco-friendly material, which can replace antibiotics that may act indiscriminately and cause ecosystem disturbance.
4. CONCLUSION
The purpose of this study was to evaluate the possibility of substituting essential oil, a natural product, for antibiotics used to treat infectious fish diseases caused by bacteria to compensate for side effects such as the emergence of resistant strains, which have been pointed out as a disadvantage of using antibiotics. We evaluated antibacterial activity of the five essential oils extracted from the leaves of pine family, and noticed that A. holophylla and P. thunbergii leaves essential oils showed strong growth inhibitory effects against gram-negative fish pathogens, E. tarda and P. damselae. As a result of analyzing the components of these two essential oils by GC-MS, they mainly consisted of monoterpene-based compounds, and the main components were identified as (-)-bornyl acetate (29.45%) and α-pinene (59.81%), repectively. In addition, we found three compounds: neryl acetate, (-)-borneol, and (-)-carveol, which are oxygenated monoterpenes. These compounds exist a very small amount but exhibit the same efficacy as essential oils. We expect that these findings will effectively control gram-negative fish pathogens by utilizing essential oils extracted from Pinaceae leaves, which are natural sources. However, in order to increase the utilization of essential oils, which are fat-soluble compounds, it is considered that studies on formulations must be conducted in advance. In addition, we anticipate that it will contribute in part to solving the problem of occurrence of resistant strains in high density fish farming due to the use of antibiotics by adding essential oils to feed additives, water quality improver, etc.