1. INTRODUCTION
Antioxidants help organisms deal with oxidative stress caused by free radical damage. Free radicals are a chemical species containing one or more unpaired electrons due to which they are highly unstable and cause damage to other molecules by extracting electrons from them to attain stability (Ali et al., 2008). Recent investigations have shown that antioxidants of plant origin having free-radical scavenging properties could have potential as therapeutic agents in the aging process and in treating free radical-mediated diseases such as neurodegeneration (Singh and Singh, 1986; Singh and Singh, 1980). Synthetic antioxidants, including butylated hydroxyanisole (BHA) and butylated hydroxytolune (BHT), have restricted use in foods because these synthetic antioxidants are suspected to be carcinogenic (Madhavi and Salunkhe, 1995). Many synthetic antioxidant compounds have shown toxic and/or mutagenic effects, which has stimulated research into natural and effective antioxidant alternatives (Nagulendran et al., 2007). Certain plant extracts and products, including flavonoids and other polyphenolic constituents, have been reported as effective radical scavengers and inhibitors of lipid peroxidation (Ohira et al., 1998; Sonwa and König, 2001).
However, many antioxidative phenolic compounds in plants are predominantly present in a covalently bound form with an insoluble polymer (Niwa and Miyachi, 1986). Therefore, it is necessary to find an effective processing method to release these compounds. Several methods, including heat treatment (steam explosion and torrefaction), far-infrared (FIR) radiation, and enzymatic treatment, have been studied in order to liberate and activate low-molecular-weight antioxidants from various plants (Gong et al., 2012; Ahajji et al., 2009; Lee et al., 2003; Duh et al., 2001; Jung et al., 2016).
Heat treatment of wood at moderate temperatures (200~250°C) under an inert atmosphere (to avoid oxidative degradation) leads to formation of torrefied or heat-treated wood (Ahajji et al., 2009). A recent study of the impact of torrefaction on lignocellulosic structures confirmed that mild torrefaction is a promising operation in the pretreatment of biomass for biofuel production (Chen and Kuo, 2010).
Additionally, biomass torrefaction: 1) improves the crushability of fibrous materials; 2) reduces the energy demand for material crushing prior to hydrolysis; 3) decomposes the biomass structure; and 4) improves the accessibility of enzymes for hydrolysis (Chiaramonti et al., 2011). This process lowers the water content of the biomass, and increases the energy density and crushability of the material (Repellin et al., 2010; Arias et al., 2008; Sheikh et al., 2013).
Antioxidative phenolic compounds can be present in raw materials or produced during the heat-treatment process. In this temperature range (200~250°C), hemicelluloses are the most affected macromolecules in wood: at 180°C, depolymerization of these components is accompanied by the release of low-molecular-weight compounds, including acetic acid, furfural, methanol, and CO2 (Avat, 1989). Previous studies have shown that the heat treatment of wood increases antioxidant activity; following torrefaction, the concentration of certain phenolic compounds, including syringyl and guaiacyl, increased (Ahajji et al., 2009; Khazraie Shoulaifar et al., 2014).
The purpose of the present study was to determine the antioxidant activity of torrefied oak wood extracts using their free radical scavenging property. Additionally, we investigated the feasibility of using these extracts with antioxidant activities as a dietary supplement for poultry.
2. MATERIALS and METHODS
Torrefaction of the oak wood (Qercus variabilis Blume) chips was achieved using a laboratory scale reactor. A prescribed amount of oak wood chips was weighed and placed in the center of the reactor. An external dryer was used to dry the chips to <15% moisture prior to torrefaction. The oak wood chips were torrefied at 240°C temperatures for 24 h. After torrefaction, the material was ground and sieved to a maximum particle size of 60 mesh. The torrefied material was stored in a desiccator at room temperature until chemical analysis. The phenolic compounds were isolated from the extracts following previously described methods, with certain modifications (Gong et al., 2012). Extractions of torrefied oak wood were performed in bottles using water. In general, an amount of 1.5, 3, 6, 12, 25g of torrefied oak wood was placed in a bottle with 100mL of water. Upon completion of extraction, the extract was filtered through a filter paper.
The total polyphenol content was determined by the Folin–Ciocalteu method according to the reported procedure (Li et al., 2008). 1 mL extracts with proper dilution were mixed with 1 mL of Folin–Ciocalteu reagent. Then, an amount of 1 mL of saturated Na2CO3 solution was added, and the mixture was allowed to stand for 1 h. The absorbance of the reaction mixture at 765 nm was measured by using a spectrophotometer (Humas HS-3300, Daejeon, Korea). A calibration curve of gallic acid was prepared, and the total polyphenol content was standardized against gallic acid and expressed as mg GAE equivalent per gram of dry torrefied wood.
The total flavonoid content was determined by aluminum chloride colorimetric assay (Abeysinghe et al., 2007). Briefly, 1 mL of extract was mixed with 10 mL of diethylene glycol, and 1 mL of 1 N NaOH and left at room temperature for 1 h. The absorbance of the reaction mixture at 420 nm was measured by using a spectrophotometer (Humas HS-3300, Daejeon, Korea). The total flavonoid content was calculated as quercetin from a calibration curve. A calibration curve of quercetin was prepared, and the total flavonoid content was standardized against quercetin and expressed as mg QE equivalent per gram of dry torrefied wood.
The DPPH radical scavenging activity was calculated based on the change of absorbance due to the decrease in 2,2-diphenyl-1-picrylhydrazyl (DPPH) in relation to the control value (Brand-Williams et al., 1995). Considering the color of the extract, an ethanol solution (1 mL) was used as a color blank instead of 0.5 mM DPPH in ethanol (1 mL). As the control, water or ethanol (1 mL) was added instead of the extract. In the following, a 0.1 mM ethanolic DPPH solution was prepared. The initial absorbance of the DPPH in ethanol was measured at 517 nm, and it did not change throughout the period of the assay. 1mL extract was mixed with 4 mL of ethanolic DPPH solution and incubated for 30 min at room temperature in the dark. Then, the absorbance at 517 nm was measured. The DPPH radical scavenging activity (%) was calculated by the following equation:
where A sample is the absorbance in the presence of the sample, and A control is the absorbance in the absence of the sample.
The reducing power was determined according to the method of Oyaizu (1986). Various concentrations of extract (0.5 mL) was mixed with 2.5 ml of 0.2 mol/L sodium phosphate buffer (pH 6.6) and 2.5 mL of 1% potassium ferricyanide. The mixture was incubated at 50 °C for 20 min. After 2.5 mL of 10% trichloroacetic acid (TCA) (w/v) were added, the mixture was centrifuged at 650 rpm for 10 min. The upper layer (2.5 mL) was mixed with 2.5 mL deionised water and 0.5 mL of 0.1% of ferric chloride, and the absorbance was measured at 700 nm: higher absorbance indicates higher reducing power. The assays were carried out in triplicate and the results are expressed as mean values ± standard deviations.
For ABTS [2,2'-azino-bis(3-ethylbenzothiazoline-6- sulphonic acid)] assay, the procedure followed the method of Re et al. (1999) with some modifications. The stock solutions included 7.4 mM ABTS+ solution and 2.6 mM potassium persulfate solution. The working solution was then prepared by mixing the two stock solutions in equal quantities and allowing them to react for 12 h at room temperature in the dark. The solution was then diluted by mixing 1 mL ABTS+ solution with 60 mL methanol to obtain an absorbance of 1.1 ± 0.02 units at 734 nm using the spectrophotometer. Fresh ABTS+ solution was prepared for each assay. Extracts (150 uL) were allowed to react with 2850 uL of the ABTS+ solution for 2 h in a dark condition. Then the absorbance was taken at 734 nm using the spectrophotometer.
The ferric reducing power of plant extracts was determined using a modified version of the FRAP (Ferric reducing antioxidant potential) assay (Benzie and Strain, 1996). This method is based on the reduction, at low pH, of a colorless ferric complex (Fe3+-tripyridyltriazine) to a blue-colored ferrous complex (Fe2+- tripyridyltriazine) by the action of electrondonating antioxidants. The reduction is monitored by measuring the change of absorbance at 593 nm. The working FRAP reagent was prepared daily by mixing 10 volumes of 300 mM acetate buffer, pH 3.6, with 1 volume of 10 mM TPTZ (2,4,6-tri (2-pyridyl)-striazine) in 40 mM hydrochloric acid and with 1 volume of 20 mM ferric chloride. All solutions were used on the day of preparation. One hundred microliters of sample solutions and 300 uL of deionized water were added to 3 mL of freshly prepared FRAP reagent. The reaction mixture was incubated for 30 min at 37°C in a water bath. Then, the absorbance of the samples was measured at 593 nm. A sample blank reading using acetate buffer was also taken. The difference between sample absorbance and blank absorbance was calculated and used to calculate the FRAP value. In this assay, the reducing capacity of the plant extracts tested was calculated with reference to the reaction signal given by a Fe2+ solution. FRAP values were expressed as mmol Fe2+/g of sample.
All experiments were repeated three times, and results are expressed as the mean ± standard deviation. Statistical analysis was performed using SPSS software, and statistical significance of the extract antioxidative effects was determined at 95% using the Duncan’s multiple range test.
3. RESULTS and DISCUSSION
Plant phenolic compounds have various physiological functions, including antimutagenic, cholesterol-lowering, anticancer, and antioxidant effects. Phenolic hydroxyl groups in the molecule bind to other macromolecules, including enzyme, It is known that it is recognized as a subject showing such a physiological activity function (Jung, 2004). Regarding the antioxidative actions, various oxygen free radical reactions in vivo are associated with aging and/or disease in living tissues. The hydroxyl group of the phenolic compound is a free radical receptor of fat, thus inhibiting oxidation (Ahn et al., 1996). Phenolic compounds were therefore extracted from the samples under the aforementioned conditions and their contents were measured. The total polyphenol content of the extract was measured using a gallic acid standard curve. The results are shown in Fig. 1. The total polyphenol contents of the extracts were 0.16, 0.26, 0.36, 0.58 and 1.07 mg/mL, respectively which were lower than those of the untreated oak wood extracts, as well as those of other treatments (Nam et al., 2014).
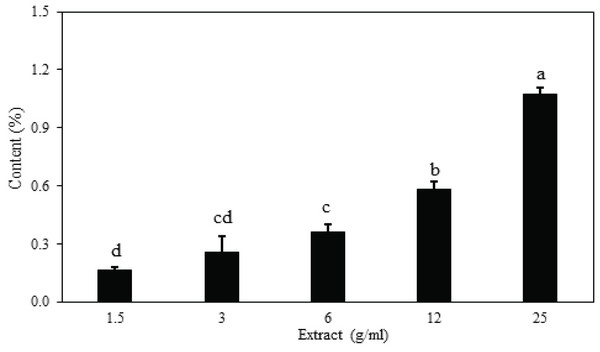
Flavonoids are the most common sub-group of the polyphenols, have c6-c3-c6 as a basic skeleton, and are yellow or light yellow in color. Flavonoids are widely distributed in nature and are known to be abundant in vegetables, grains, and fruits including the stems and roots (Hertog et al., 1993). Flavonoids have high antioxidant abilities, effectively eliminating all reactive oxygen species, as well as antiviral, anti-inflammatory, and anticancer effects, similar to other polyphenols (Tsao, 2010). The total flavonoid content of the extracts was measured using a quercetin standard curve, and the results are shown in Fig. 2. The total polyphenol contents of the extracts were 4.64, 15.09, 40.32, 85.36 and 192.12 mg/mL, respectively. The extract polyphenol contents were lower than those of the oak extracts, but the flavonoid content was higher.
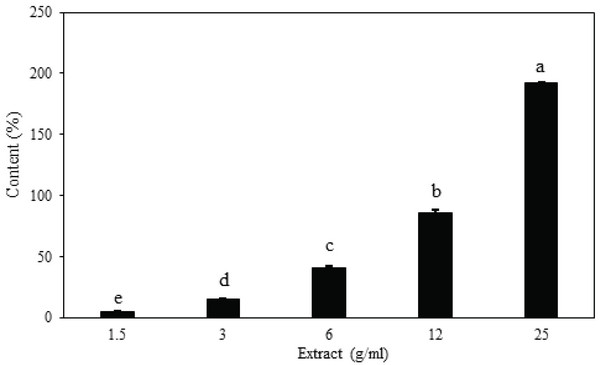
1,1-Diphenyl-2-picrylhydrazyl (DPPH) is relatively stable compared with the highly reactive superoxide and hydroxyl species that are primarily responsible for oxidative damage in biological systems. It is widely used to test the ability of compounds to act as free radical scavengers or hydrogen donors. The DPPH molecule is considered a model for a lipophilic radical. A certain chain in lipophilic radicals is initiated by lipid auto-oxidation (Cotelle et al., 1996; von Gadow et al., 1997; Soare et al., 1997). DPPH is a stable free radical at room temperature, and accepts an electron or hydrogen to become a stable diamagnetic molecule (Chen and Ho, 1995). The reduction capability of DPPH was determined by the decrease in its absorbance at 517 nm, which is induced by antioxidants. A positive DPPH test suggests that the samples were free radical scavengers. Varied extract concentrations (1.5, 3, 6, 12 and 25 g/mL) showed that antioxidant activities were dose-dependent (47.20, 74.78, 87.41, 87.19 and 85.34%, respectively) in the DPPH radical scavenging assay. The antioxidant activities of each extract and extract concentration was compared using the differences in the reference densities. These results suggest that the extracts are valuable natural resources with excellent antioxidant ability. Fig. 3.
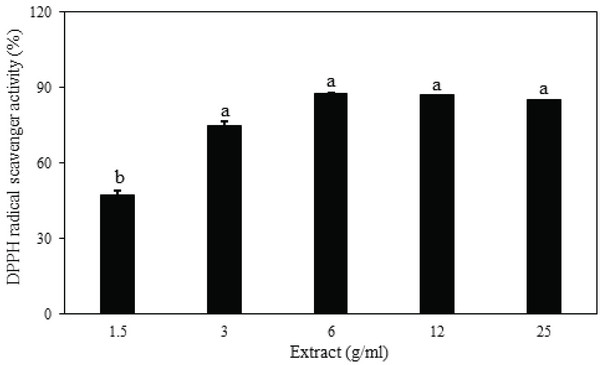
Ferrous ions (Fe2+) was measured spectrophotometrically at 700 nm, with higher absorbance values indicative of greater reducing capacity of ferric (Fe3+) to ferrous (Fe2+) ions (Lue et al., 2010). It is considered that the higher the absorbance value, the better the reducing power. The reducing activity of extracts of torrefied oak wood. Moieties with reducing power show that they are electron donors, and they can reduce the oxidized intermediates so they can act as antioxidants. As with the antioxidant activity, the reducing power of the water extract from torrefied oak wood was also concentration-dependent. Hence, the reducing power of the extract is increased as the concentration is increased. The reducing power of the 25 g/mL extract was significantly higher than that of the 1.5, 3, and 6 g/mL extracts, but there was no significant difference between the 12.5 and 25 g/mL extracts (p > 0.05). Fig. 4.
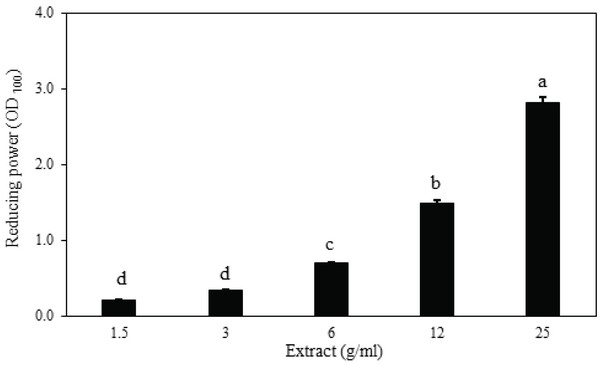
DPPH and ABTS assays are widely used to determine the radical scavenging ability of various samples (Marowicz et al., 2004; Jang et al., 2007; Bursal and Gülçin, 2011). The DPPH assay is useful for determination of the free radical scavenging ability of extracts; the ABTS radical decay assay can be used to measure the kinetics of the free radical scavenging power of extracts. ABTS radicals are more reactive than DPPH radicals, and, unlike the reactions with DPPH, the reactions with ABTS radicals generally occur in less than a millisecond (Naik et al., 2003). ABTS radical cation decolorization can measure both hydrogen-donating antioxidants and chain-breaking antioxidants, and it can be applied to both the aqueous and the organic phases. ABTS+ free radicals generated by a reaction with potassium persulfate can be removed by antioxidants in the extract. It is radically discordant to discoloration of a specific index of cyan. (Leong and Shui, 2002; Aktumsek et al., 2013). ABTS is generally used for testing the preliminary radical scavenging activity of antioxidant compounds and/or plant extracts. The ABTS scavenging capacity of plant extracts was expressed as an inhibition capacity, and the results are shown in Fig. 5. ABTS radical scavenging activity tended to increase in a dose-dependent manner, similar to that of the free radical scavenging activity. The assay provided measurements of 0.25 ± 0.01 at a 6 g/mL concentration, and 0.27 ± 0.03 for the 25 g/mL extract. In addition, the polyphenol and ABTS contents were low, but the phenolic compound profile associated with the antioxidant activity may still be contained within the extract.
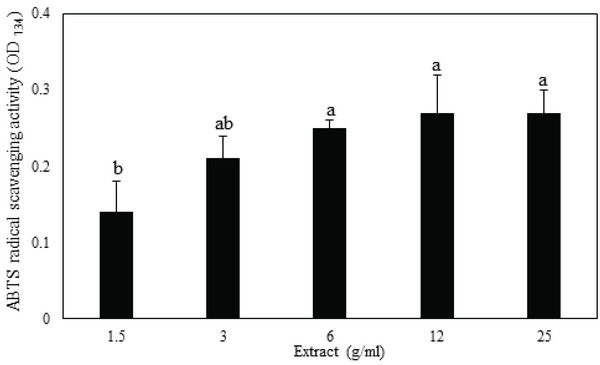
The FRAP assay is based on the ability of an antioxidant to reduce Fe3+ to Fe2+ in the presence of TPTZ, forming an intense blue Fe2+- TPTZ complex with an absorption maximum at 593 nm. This reaction is pH-dependent. The absorbance value decrease is proportional to the antioxidant content of the extract (Liu et al., 2009). Studies have indicated that the antioxidant effect is associated with the development of reductones (Yen and Duh, 1993). Briefly, reductones are reported to be terminators of free radical chain reactions; thus, the antioxidant activity of an aqueous extract may be associated with its reductive activity (Gordon, 1990; Dorman et al., 2003). The antioxidant activity of FRAP was increased accordingly with increases in the extract concentration. As shown in the figure, FRAP activity was determined to be 0.44 ± 0.03, 0.76 ± 0.01, 1.49 ± 0.02, 2.70 ± 0.03, and 2.77 ± 0.04 at extract concentrations of 1.5, 3, 6, 12.5, and 25 g/ml, respectively. In the case of FRAP activity, it is presumed that the specific color of the sample affected the experimental data. Fig. 6.
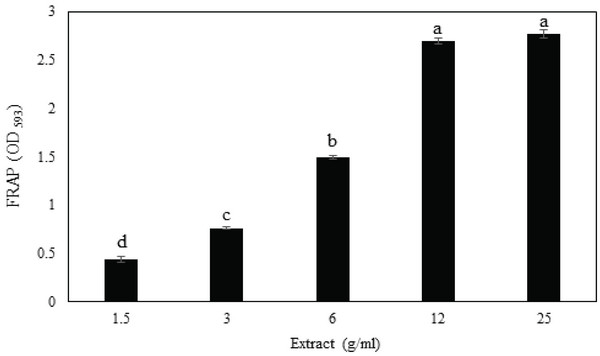
To correlate the results obtained with the different methods, a regression analysis was performed (correlation coefficient (R), Table 1). Significant correlations were found between the various methods used to determine the antioxidant potential, especially between DPPH and ABTS assays (R = 0.9505), and ABTS and FRAP assays (R = 0.7859). The lowest correlations were found between the FRAP and DPPH assays (R = 0.5812). The lowest correlations were found between the FRAP and DPPH assays (R = 0.5812).
TP | TF | DPPH | ABTS | |
---|---|---|---|---|
TF | 0.9973 | |||
DPPH | 0.3538 | 0.3139 | ||
ABTS | 0.5408 | 0.5001 | 0.9505 | |
FRAP | 0.7718 | 0.7542 | 0.5812 | 0.7859 |
The correlation between total polyphenol contents and DPPH of extracts had a correlation coefficient of R = 0.3538.
A strong correlation was found between the ferric reducing potential, determined by the FRAP assay, and total polyphenolic content (R = 0.7718). It can be concluded that antioxidant activity of plant extracts is not limited to phenolics. These results indicate a relationship between phenolic compound concentration in plant extracts and their free radical scavenging and ferric reducing capacities. Therefore, the presence of phenolic compounds in plant extracts contributes significantly to their antioxidant potential. This result is in agreement with previous reports that ferric reducing potential can be related to phenolic content (Katalinic et al., 2006; Wong et al., 2006; Dudonné et al., 2009).
4. CONCLUSION
The antioxidant activity of the extracts extracted by the cold water extraction method was evaluated to evaluate the applicability of the water extract from torrefied oak wood as an antioxidant. The DPPH radical scavenging activity was evaluated as a high radical scavenging activity of more than 80% at 12 g/mL. As a result of the evaluation of FRAP activity, DPPH radical scavenging activity showed excellent activity. Reducing power and ABTS activity of the water extract from torrefied oak wood were dose dependent. ABTS activity was slightly lower than that of radical scavenging activity and FRAP activity. Therefore, the antioxidative effect of the water extract from torrefied oak wood is thought to be due to its high radical scavenging activity and high reducing power. The antioxidant activity of antioxidant activity of antioxidant activity was correlated with that of phenolic compounds. It is considered that the water extract from torrefied oak wood contains antioxidant activity by containing phenolic compounds. Therefore, the use of a small amount of the extract for the dietary supplementation of poultry is predicted to convey the benefits of the reported multiple antioxidant activities (Puthpongsiriporn et al., 2001; Mielnik et al., 2006; Luber et al., 2003), and hence have a positive effect on the productivity of breeding poultry.