1. INTRODUCTION
Solid wood thermal modification has been widely studied over the years (Hill, 2006). In general, thermal modification reduced hygroscopicity and water absorption, thus increasing dimensional stability and decay resistance. However, the information on changes in sound absorption property and permeability of wood after high temperature heating treatment was scare.
Malas wood has been used as interior or exterior materials because of its good hardness, resistance to weathering and relatively good elastic properties. In the case of the wood used as interior materials, the proper sound absorption capability of wood was necessary for good acoustic environment. It was recognized that the sound absorption capability of wood was influenced by airflow resistivity or air permeability that varied according to the sound incident surface structure and pore characteristics (kang et al., 2012; kang et al., 2008; kang et al., 2010; kang et al., 2011; wassilieff, 1996).
Therefore, the purpose of this study was to evaluate effect of high temperature heating treatment on the sound absorption property and permeability of malas wood which was one of widely used wood in southeast Asia.
2. MATERIALS and METHODS
Malas (Homalium foetidum) lumbers imported from Papua New Guinea were used in this study. The green malas lumbers with 120 mm in width, 30 mm in thickness and 1800 mm in length were heated under the temperature of 190°C for 3 hours. From green malas and heat treated malas, five cylindrical log cross sections with 28.9 mm in diameter and 10 mm in thickness were prepared. The total quantity of 10 pieces log cross sections were oven dried under the temperature of 100°C. The average moisture content (MC) of control and heat treated specimens were 6.08% and 4.12%, respectively. The average specific gravity of control and heat treated specimens were 0.76 g/cm3 and 0.65 g/cm3, respectively.
The permeability of specimens was measured by membrane pore size analyzer (Porous Material Incorporated, CFP-1200AEL) according to capillary flow porometry as precious research (kang et al., 2017). The principle of this method was described in ASTM 316-03. The pore structure and permeability were evaluated using liquid and gas flow as a function and the pressure difference. When a wood specimen was soaked in the wet solution, the specimen with different diameter of holes was easily wet because the solution had the low surface tension of 15.9 N/m. After thoroughly soaked specimen was put into sample chamber, the chamber was sealed as shown in Fig. 1, and then non-reactive nitrogen gas was circulated. The bubble point was first measured at the pore with largest diameter inside which the pressure was the minimum value compared to other pores, that is, at the place to overcome the capillary action of the fluid from gas pressure, as the solution was forcing out. After that, the flow was measuring until all pores was empty as the pressure being increasing. The pressure and flow rate of the gas through the dried specimen were measured although the liquid in all pores was not existence. The gas permeability and pore structure of wood specimen were evaluated from the results. The gas permeability of wood specimen was calculated according to Equations (1) and (2).
where, v = flow rate, k = permeability, μ = viscosity, p = pressure, x = location
where, F = flow, A = cross sectional areas
The sound absorption rate of specimen was measured by two microphone transfer function method using impedance tube (B&K company, kit type 4706), pulse analysis equipment and a spectrum analyzer (B&K company), as precious research (kang et al., 2012). In this study, the variation of sound absorption rate was measured as change of frequency in the range of 50~6,400 Hz. During measurement, temperature, relative humidity and atmospheric pressure were 25°C, 52% and 1008.0 hPa for control specimens and 24.6°C, 48% and 1008.0 hPa for treated specimens, respectively. Moreover, the velocity of sound, air density and acoustic impedance were 346.15 m/s, 1.176 kg/m3 and 407.0 Pa/ (m/s) for control specimens and 345.92 m/s, 1.177 kg/m3 and 407.2 Pa/(m/s) for treated specimens, respectively.
3. RESULTS and DISCUSSION
Capillary flow porometry estimates the constricted pore diameter of a porous material, which is the smallest pore diameter of one flow pathway. This constricted pore may be enlarged by heat treatment as shown in Fig. 2. The pore size distribution as a function of average pore diameter by CFP represent that the pore diameter enlarged and the number of permeable pores in treated wood increased, as compared to control wood.
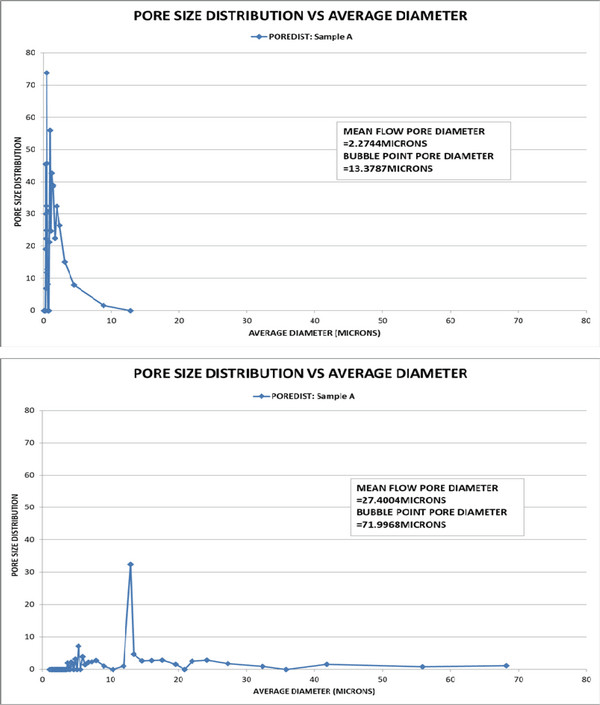
The change of permeability for control and treated specimens is presented in Fig. 3. Average permeability of control specimens was 3.11 darcy while that of treated specimens was 23.48 darcy, which is about 8 times higher than that of control specimens. This trend could be attributed to the flow passageway inside wood after treatment being more and larger than before treatment.
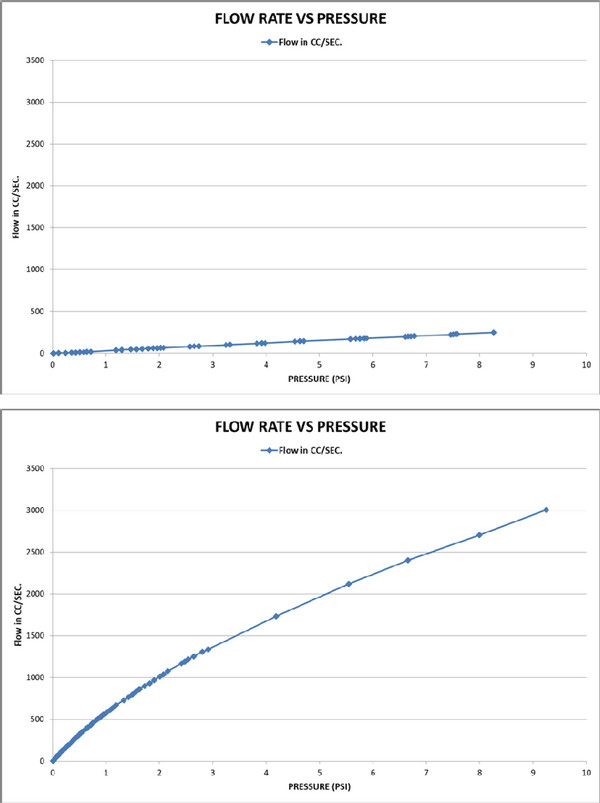
The sound absorption rates of specimens in the frequency range of 50~6,400 Hz are shown in Fig. 4.
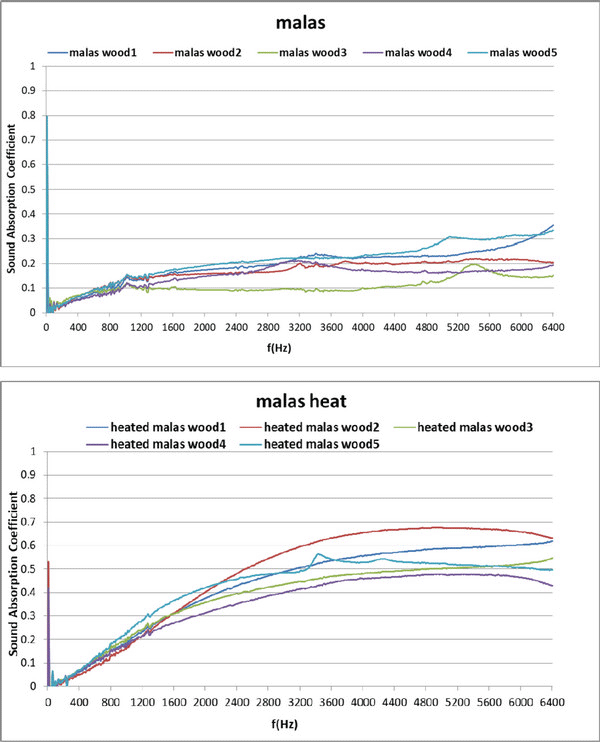
As showed in Fig. 4, the sound absorption rates of treated specimens showed higher values than those of control specimens in almost all frequency range. The difference in sound absorption rates between treated and control specimens became larger with increasing of frequency beginning from 1 kHz, reached 2 times in frequency range of nearby 2 kHz and nearby 6 kHz. It indicates that the sound absorption rate of treated specimens is increasing with increasing of frequency, which is a typical characteristic of porous sound absorber. This trend could be accounted for there being more pores and larger flow passageway in wood due to the inclusions inside wood such as gum, carbohydrate, etc. being melted and discomposed out during treatment. It can be inferred that the sound absorption rate of treated specimens was significantly increased because the flow of the air as the medium transferring the energy of sound wave was increased due to improved permeability during heat treatment.
The NRC (noise reduction coefficient, mean value of sound absorption coefficient at the frequency of 250, 500, 1,000 and 2,000 Hz) showed a value of 0.17 for treated specimens and 0.10 for control specimens, which indicated that the NRC of treated specimens is improved by 70 percent compared to that of control specimens. It is advised that to apply the heated malas wood as environmentally friendly sound absorption materials is possible.
4. CONCLUSION
In order to evaluate changes in sound absorption property and permeability, the sound absorption rate and permeability of heated malas wood were measured before and after heat treatment in this study. The results of this study were as follows:
The sound absorption rate of treated specimens showed high value in almost all frequency range. Thus malas wood after heat treatment was could be considered as sound-absorbing materials because its mean value of sound absorption rate in the frequency range of 50~6400 Hz was over 40%.
The permeability of heated malas wood was increased by about 8 times compared to that of control.
The pore diameter of heated malas wood was enlarged compared to that of control.