1. INTRODUCTION
Urea-formaldehyde (UF) resins are widely used worldwide because they have high reactivity, good adhesion, and are inexpensive. However, because of their low water resistance, UF resins are not stable in a humid environment and the emission of formaldehyde released from cured UF resins is harmful. Meanwhile, melamine-urea-formaldehyde (MUF) resins were born to solve the problem of UF resin and took advantage of melamine. MUF resins are thermosetting resins made by reacting urea, melamine and formaldehyde together, and have the advantage of having better water resistance than UF resin, lower amount of formaldehyde emission, and colorless, and have the advantage of being cheaper and phenol resin (Jeong et al., 2019; Pizzi, 2003). The MUF resins are more stable in binding to methylene of the amide group from the triazine ring of melamine than UF resins, and thus more resistant to water (Dunky M, 1998). Because of their good adhesion strength and less formaldehyde emission, MUF resins have been especially used for the production of exterior grade wood-based panels (Tohmura et al., 2001, Zhou et al., 2012). For example, it was found that melamine can be added to urea resin in a minimum amount to increase water resistance and reduce the amount of formaldehyde emission by C-NMR spectroscopy (Dunky M, 1998; Mercer and Pizzi, 1996).
In general, the adhesion performance of an adhesive is determined by the degree of penetration into the porous wood of the interconnected cells and the bonding strength (Kamke and Lee, 2007). The factors that determine the penetration power of an adhesive into wood are the chemical structure, molecular weight (MW), molecular weight distribution (MWD), additives, solids, viscosity, curing time, buffering capacity, hardening time, curing speed, contact angle (Kamke and Lee, 2007;Shi and Gardner, 2001). Among them, MW and MWD have an important influence on the performance of an adhesive. If the MW is low, it penetrates into the wood cell wall, but the adhesive layer is thin (Hunt et al., 2018; Kamke and Lee, 2007; Roh, 1999).
The properties and performance of these MUF resins depends on their synthesis method such as an addition order, molar ratio, and melamine content (Pizzi et al., 2003; Hunt et al., 2018; Jeong and Park, 2019; Lubis and Park, 2020). For example, the MW of MUF resins in the multi-step reaction synthesis was higher than that of the simultaneous reaction synthesis (Tohmura et al., 2001). In addition, the MUF resins prepared by reacting hydroxymethylated melamine with urea was better than the MUF resins synthesized by simultaneous reaction of melamine, urea and formaldehyde, regardless of the type of curing agent (Roh, 1999). There was also a study using a synthetic method in which the F/U molar ratio was set differently in the hydroxymethylation and condensation steps according to the order in which urea was added (Paiva et al., 2012). And it was reported that the resin with the highest F/U molar ratio had significantly low MW molecules and more polymers with high MW in the gel permeation chromatography (GPC) analysis (Paiva et al., 2012).
Similar to the synthesis method of MUF resins, the melamine content clearly affects the gel time, solid content, and viscosity (Hong et al., 2017). Their result showed that the reactivity of the MUF resins increased and thus the gel time decreased as the melamine content increased, regardless of the synthesis method (Hong et al., 2017). In addition, thinner bond-line thickness and greater resin penetration in plywood were obtained with MUF resins synthesized by reacting melamine and formaldehyde simultaneously followed by urea (Lubis et al., 2019). In contrast, MUF resins synthesized in two-batch method increased viscosity and decreased solid content as melamine content increased (Hong et al., 2017). In addition to these properties, the melamine content also affects the chemical properties. As the melamine content increased, the crystallinity was amorphous because of the three-dimensional network formed by the increase of the linkages between the melamine molecules with an increase of branched methylene bonds (Tohmura et al., 2001). Accordingly, the adhesion strength improved with an increase of cohesion. Therefore, the melamine content affects the MW, curing, gelation time, interphase, and adhesion of MDF (Tohmura et al., 2001). As the melamine content increased, the MW increased and the high MW portion increased, the low MW portion decreased (Tohmura et al., 2001). As the melamine content increases and the MW increases. So, the resin penetration depth decreases and the bond line thickness increases (Jeong and Park, 2016; Nuryawan et al., 2014). If necessary, a resin can be prepared by adjusting the melamine content to obtain a certain target molecular weight.
To analyze the MW of MUF resin, the GPC had been used in many studies (Jeong and Park, 2016) (Jeong et al., 2019) GPC is a detector that uses the phenomenon of separating substances by MW difference using a porous stationary phase inside. The molecular weight distribution of GPC is calculated from the calibration plot of standard materials. At the constant flow of mobile phase, high MW species in the sample come out quickly and the low MW species slowly release because of the pore size of the packed porous material inside the column of GPC. Although it is not possible to measure the absolute MW, GPC parameters such as flow rate, column-detector temperature and sample injection temperature affects the MW of UF resins (Jeong and Park, 2017). They showed that the number average molecular weight (Mn), weight average molecular weight (Mw), and polydispersity index (PDI) decreased as the flow rate increased. As the column-detector temperature increased the sample temperature increased, then the Mn, Mw, and PDI increased. In addition, when the flow rate was 0.5 ml/min, column-detector temperature and sample injection temperature were 50°C, the MW had the largest value (Jeong and Park, 2017). In another study of the MWD of UF resins was affected by the degree of condensation reaction (Dunky, 1998).
GPC had been widely used to measure MW of MUF resins since the MUF resins began to attract attention. For example, Jeong et al. (2019) measured the MW of MUF resins, and they reported that as the melamine content increased, the molecular weight increased, and the adhesion of the bond line, plywood and medium density fiberboard (MDF) increased, but the crystallinity of the resin, the resin penetration depth, and the formaldehyde emission decreased. These findings reveal the effect of melamine content and synthesis method on the cohesion that determines the adhesion performance of MDF and plywood, and the effect on adhesion such as resin penetration depth and adhesive layer thickness (Jeong et al., 2019). It was also confirmed that the degree of polymerization gradually increased with the aging period of the formation and aggregation of colloidal particles of the MUF resin (Zanetti and Pizzi, 2004). It was also reported that MUF resins had various distributions with different molecular mass fractions during the reaction process (Lei et al., 2006). When the MW of MUF resins at various pH and melamine levels, the peaks with a relatively large retention volume had a small molecular weight of 3000. The peaks with a relatively small retention volume resulted in high MW of 12000 (Paiva et al., 2012).
As mentioned above, although the MW of MUF resins have been analyzed in the literature so far, the GPC parameters are all different and inconsistent. Therefore, this paper aims to investigate the effect of the synthesis method, melamine content, and GPC parameters on the MW measurement of MUF resins.
2. MATERIALS and METHODS
Formalin (37%), melamine (99%), urea granules (99%) were supplied by Daejung Chemical, Korea. Aqueous solutions of formic acid (20 wt%) and NaOH (20 wt%) was added to adjust the pH during the resin synthesis. High-purity (99.9%) N,N-dimethyl formamide (DMF) and dimethylsulfoxide (DMSO) of high-performance liquid chromatography (HPLC) grade were purchased from Sigma- Aldrich and used without further treatment.
In this study, two types MUF resins were synthesized by the previous method (Jeong et al., 2019). MUF-A resins were synthesized by simultaneous reaction of urea, melamine, and formaldehyde at the same time. The MUF-A resins were synthesized using an alkaline-acid-alkaline three-step reaction. First, about 580g of formalin was placed in a reactor equipped with a mantle heater. Then, sodium hydroxide (20 wt%) was added to adjust the pH to 8.2-8.5. The first urea and melamine (5, 10, and 20 wt%) were simultaneously reacted with formaldehyde in the reactor at a 1.5 molar ratio of F/(M+U) under a continuous stirring at 40°C. After this, the temperature was increased to 90°C for an hour. Then, in order to obtain a condensation reaction, formic acid (20 wt%) was used to adjust the pH to an acid state of 6.5-7.0. When the target viscosity of the resin is the ‘B’ and ‘C’ of the bubble viscometer (VG-9100, Gardner-Holdt Viscometer, FL, USA) at 90°C, the condensation reaction was stopped. After that, the pH was adjusted to 7.5-8.0 with 20% NaOH. Then, after adding the second urea to obtain a final F/(M+U) molar ratio of 1.0, the temperature was reduced to 60°C in 20 minutes. The resulting MUF resin was cooled to ambient temperature and adjusted to pH 9.0-9.2 before the measurements.
MUF-B resins were synthesized in a multi-step reaction of reacting melamine with formaldehyde first, and then with urea. The MUF-B resins were also synthesized using an alkaline-alkaline-acid three-step reaction. About 580 g of formalin was placed in a reactor equipped with a mantle heater. Then, as in the synthesis method of MUF-A, sodium hydroxide (20 wt%) was added to adjust the pH to 8.2-8.5. Unlike MUF-A, melamine at three contents (5, 10, and 20%) was added and continuously stirred at 40°C. Then, the temperature increased to 90°C and maintained for an hour. Then, the first urea was added to the reactor and maintained for 10 minutes while adjusting to the same 1.5 F/(M+U) molar ratio. When the viscosity of the target resin reached between the ‘B’ and ‘C’ states of the bubble viscometer, the condensation reaction occurred by adjusting to pH 6.5-7.0 with formic acid (20 wt%). And then, the condensation reaction was stopped at 100, 50, and 20% of the target water tolerance corresponding to the melamine content of 5, 10, and 20%. After the target water tolerance was reached, the pH was adjusted to 7.5-8.0 with 20% NaOH. Then, after adding the second urea to obtain a final F/(M+U) MUF resin of 1.0 molar ratio, temperature was reduced to 60°C for 20 minutes. MUF-B resins were cooled to ambient temperature and adjusted to pH 9.0-9.2 before the measurement.
A cone-plate viscometer (DV−II+, Brookfield, MA, United States) was used to measure the viscosity of MUF-A and MUF-B resins at 25°C under 60 rpm using spindle No. 2. About 1 g of the sample was dried in an oven at 105°C for 3 h to obtain the non-volatile solids content of both resins by measuring the sample mass before and after the drying. A gel time meter (DAVIS, Sunshine Instruments, United States) was used to measure the gelation time of the resins at 100°C by adding 3% NH4Cl based on the resins’ solids content. The water tolerance of MUF-A and MUF-B resins was determined by checking a clouding point after adding water into 1 g resins in the tube at 20°C until the appearance of resin changed from transparent to cloudy.
A GPC (YL9100, Younglin, Gyeonggi-do, Korea) system equipped with a refractive index (RI) detector was used for the molecular measurement. Three columns (KD 801, 802 and 806 M from SHODEX, Japan) were used. Column-detector temperatures were set differently at 35°C or 50°C. Four flow rates of 0.3, 0.5, 0.8, and 1.0 ml/min were used. HPLC grade DMF was used as the mobile phase. The MUF resin sample was dissolved in 10% DMSO and 90% DMF, and then injected into the GPC at the injection temperature at 25 or 50°C. The sample injection temperature of 25°C was done at ambient temperature. In order to obtain a sample injection temperature of 50°C, the sample was placed in an oven at 50°C for 3 hours or longer, and then injected. The sample solution was filtered through a 0.45 μm filter prior to the injection into the GPC. The weight average molecular weight (Mw), number average molecular weight (Mn) and polydispersity index (PDI)were calculated using YL-Clarity chromatography software (Younglin, Gyeonggi-do, Korea).
As presented in Table 2, three GPC parameters (flow rate, column-detector temperature, and sample injection temperature), and two synthesis variables (i.e. method and melamine content) were designed to measure MW of MUF resins. Total ninety-six measurements were made in this study. In particular, the resin synthesis variables are two synthesis methods and three melamine contents (5, 10, and 20 wt%) for MUF-A and MUF-B resins. The GPC parameters are flow rate (0.3, 0.5, 0.8, and 1.0 ml/min), column- detector temperature (35-35, and 50-50°C), and sample injection temperature (25 and 50°C).
3. RESULTS and DISCUSSIONS
As presented in Table 1, pH, viscosity and solids content of MUF-A and MUF-B resins increased as the melamine content increased, regardless of the synthesis method. And the gel time of these resins decreased. In addition, these two resins had similar non-volatile solids content and water tolerance vales, but MUF-A had a relatively higher viscosity than that of MUF-B resins. MUF-A resin had longer gel time than MUF-B resins.
Fig. 1 shows GPC chromatograms of MUF-A and MUF-B resins at four different flow rates. Regardless of the synthesis method, the chromatogram showed the first peak when the flow rate of both resins was 1.0 ml/min. As the flow rate decreased, the peak was detected later in the retention time because the sample was stayed longer at lower flow rate. When the flow rate was 0.3 ml/min, both resins had the longest retention time. As the flow rate increased to 1.0 ml/min, both resins had the shortest retention time. These indicate that a shorter retention time gives low elution volume, which could result in lower molecular weight. If the retention time is long, the detection time is longer and the elution volume increases accordingly. So, the molecules are large and the molecular weight is high. Many experiments have shown that as the flow rate decreases, the retention time and elution volume increased (Jeong and Park, 2017). Conversely, as the flow rate increases, the elution volume decreases. At higher flow rates, the elution curve is distorted due to insufficient diffusion into micro pores (Uglea, 1996). The Mw, Mn, and PDI values of 0.3 ml/min, which were the slowest in both MUF-A and MUF-B resins, had the largest values among all flow rates.
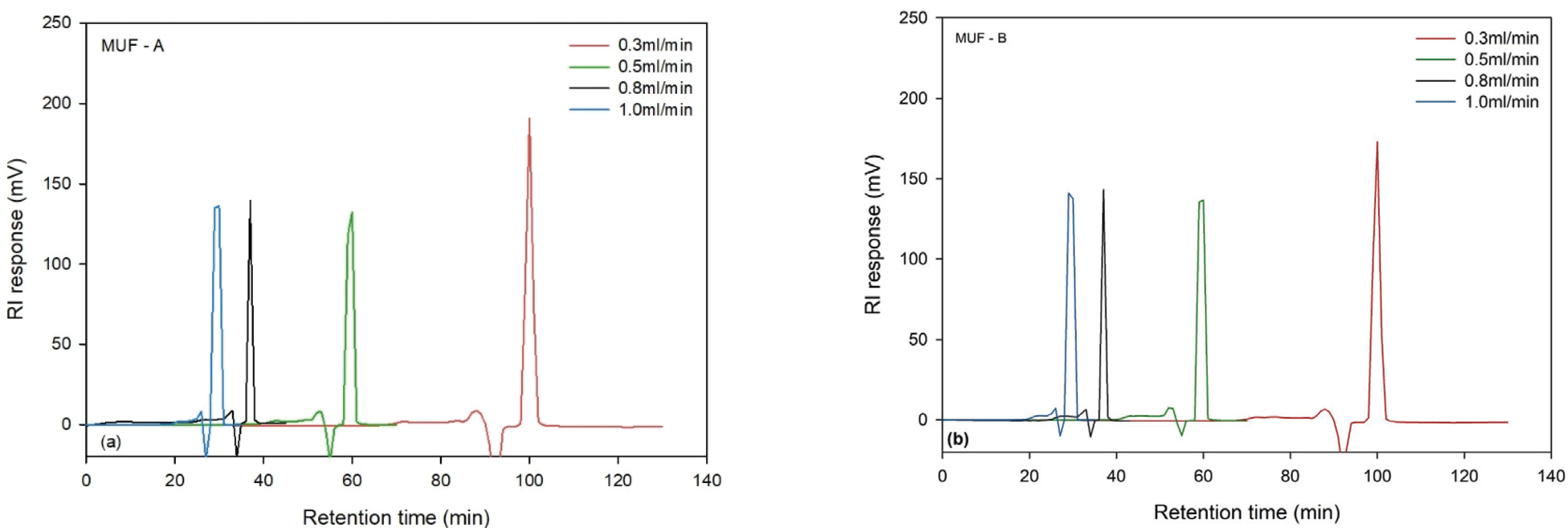
Fig. 2 displays the Mw, Mn, and PDI of two types of MUF resins prepared with different synthesis methods and melamine contents as a function of the flow rate of GPC. When the flow rate is 0.3 ml/min, the MUF-A resins with 10% melamine content show the lowest Mw, while MUF-B resins have the highest Mw. In addition, the Mw and PDI values of MUF-B resins are higher than those of MUF-A, regardless of the melamine content. MUF-A and MUF-B resins show opposite results, showing an unstable measurement of MW at this flow rate in the GPC (Fig. 2, a and b). While, unlike results of 0.3 ml/min flow rate, the MW of both MUF-A and MUF-B resins increased as the melamine content increased, and the Mw was the largest when the melamine content was 20%. And the Mw of MUF-B resins was greater than those of MUF-A resins, which could be due to a greater degree of branched polymers produced by the MUF-B resins. These results indicated that the peaks of GPC chromatograms were getting wider and higher as the melamine content increased (Jeong et al., 2020).
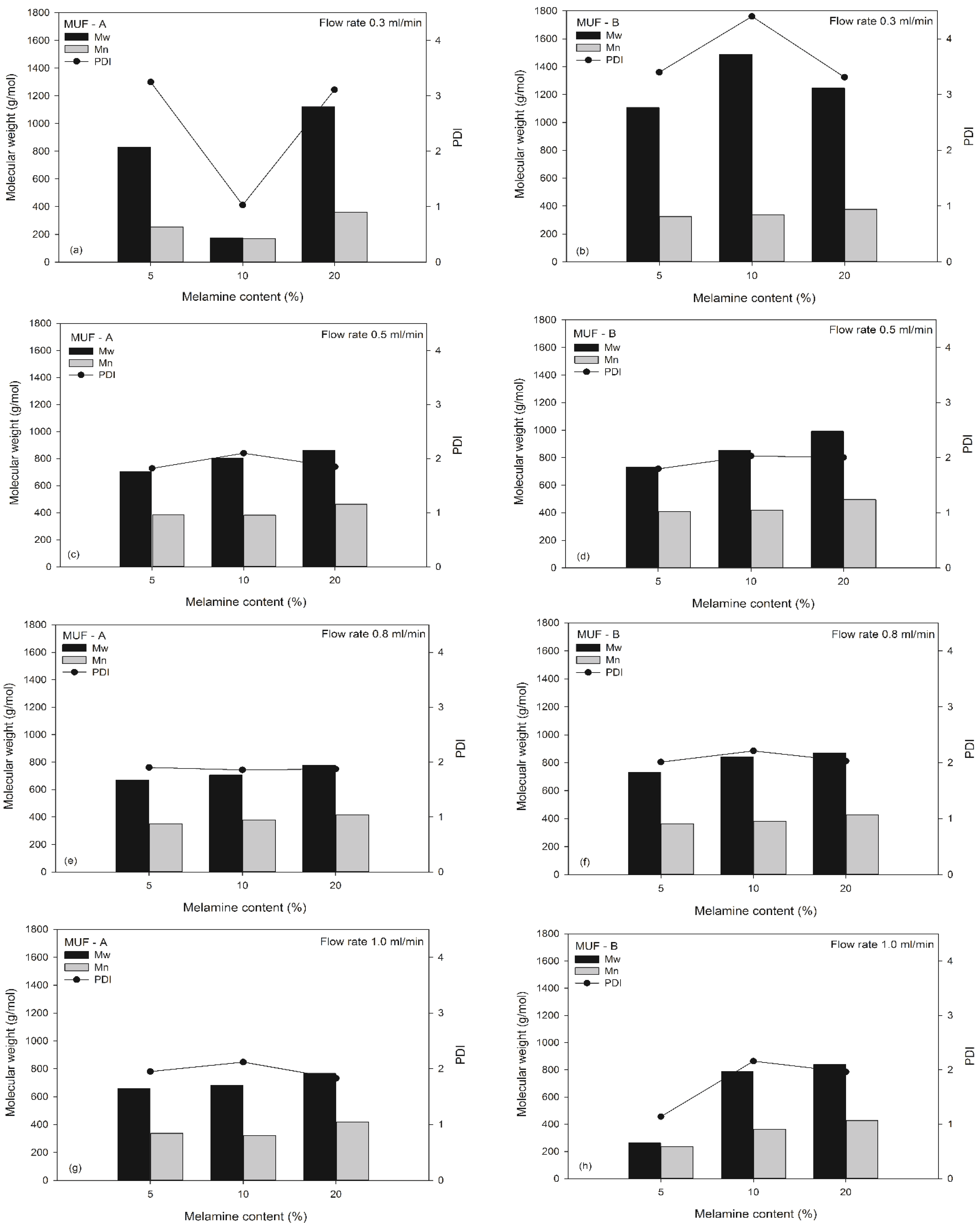
As an index of the MWD of a polymer, a higher PDI means greater MWD of a polymer. In fact, regardless of the synthesis method, it was revealed that the PDI value was highest when the melamine content was 10% at all flow rates. When the melamine content was 20%, the PDI decreased and the MWD narrowed. These results generally indicate that the MW increases as the melamine content increases. In addition, the Mw of all MUF resins was the highest at 0.5 ml/min and then gradually decreased as the flow rate increased from 0.5 to 1.0 ml/min. This result suggests that a proper flow rate for MF reins is 0.5 ml/min that can differentiate the effects of synthesis method and melamine content on the MW of these resins.
The C-D temperature was set as either 35-35°C or 50-50°C to compare its effect on the MW of MUF resins. Fig. 3 shows the MW of MUF-A resins at four flow rates according to the C-D temperatures when the sample injection temperature is either 25°C or 50°C. When the sample injection temperature and C-D temperature were 25°C and 50-50°C, the Mw of MUF-A resins was greater than that of MUF-B resins. But when the sample injection temperature and C-D temperature were 50°C and 35-35°C, the opposite result was obtained. When the C-D temperature increases to 50-50°C at the 25°C sample injection temperature, the solubility is improved as the resin molecules are more dissolved in the solvent, resulting in an increase in the MW. The reason could be attributed to a reactivity difference between urea and melamine to formaldehyde (Zhang et al., 2013). In other words, urea reacts more quickly with formaldehyde than melamine does in the simultaneous reaction for MUF-A resins.
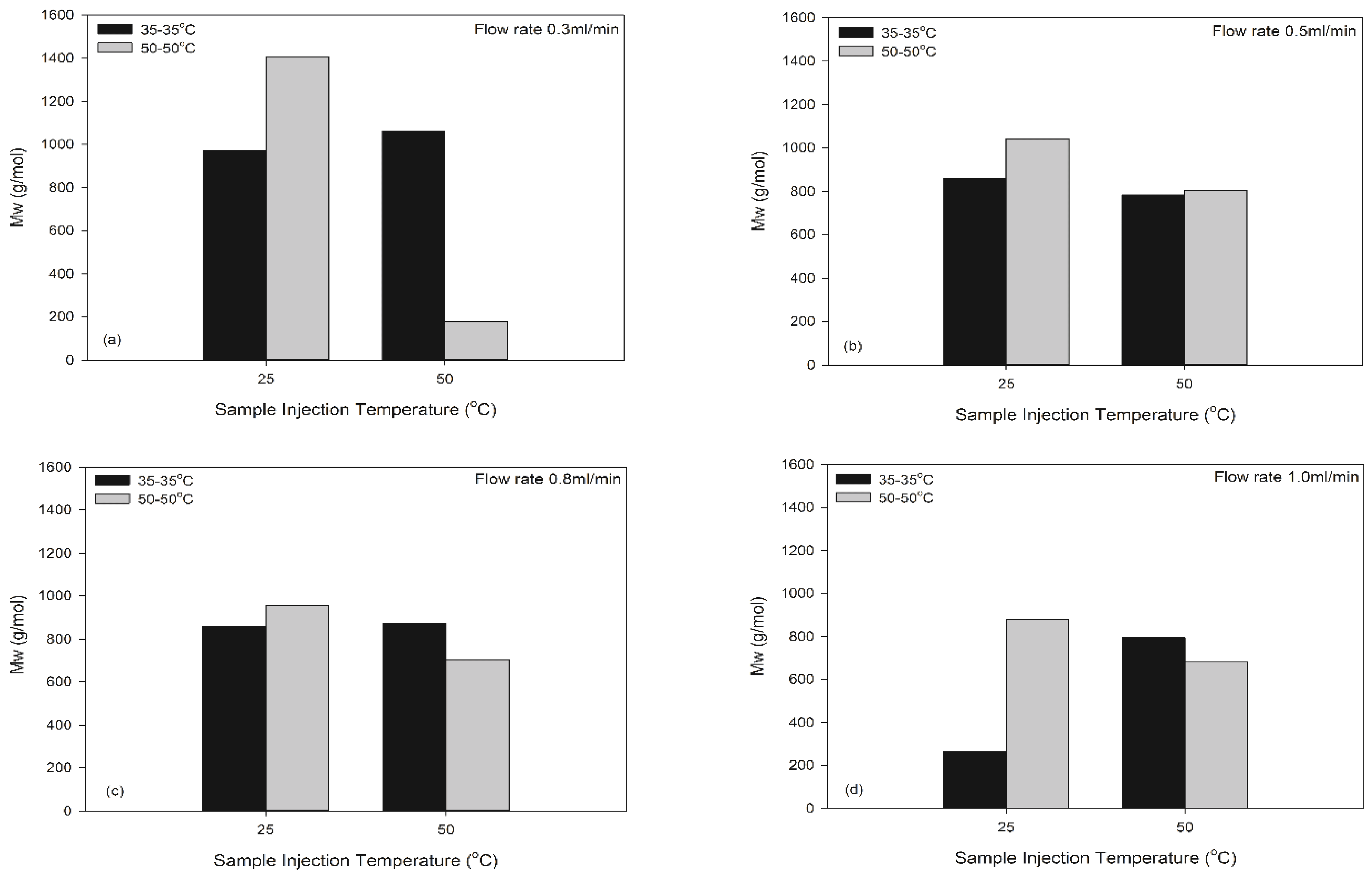
Fig. 4 presents the MW and PDI of MUF-B resins, depending on the C-D and sample injection temperatures. The PDI value and MW was highest when the melamine content was 10% for both MUF-A and MUF-B resins. Therefore, the 10% melamine of MUF resins were used to compare the MW at different C-D temperatures. The MUF-B resins had much larger Mw at the C-D temperature of 50-50°C that at 35-35°C for all flow rates except 1.0 ml/min. As the C-D temperature increased, the solubility in the column increases. This could be also due to that MUF-B resins contain less branched molecules than those of MIF-A resins. These results also suggest that the C-D temperature of 50-50°C provide a proper MW measurement of MUF resins, which is consistent with UF resins (Jeong and Park, 2017).
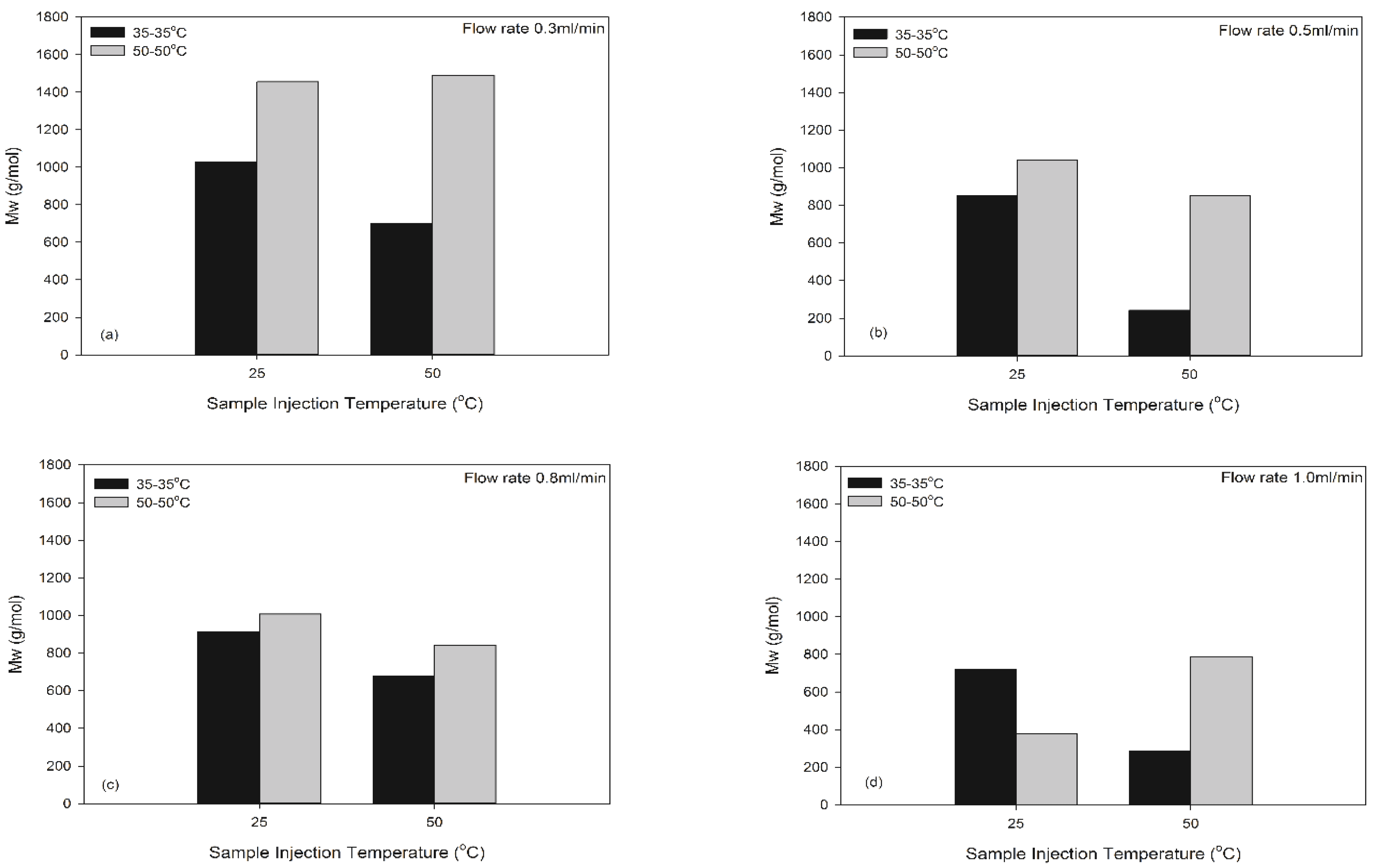
The MW of MUF resins was also compared at different sample injection temperatures at the C-D temperature of 50-50°C that provided a consistent MW measurement, and the results were shown in Fig. 5. Even if the Mw of MUF resins are not consistent depending on the sample injection temperature, larger Mw is detected at the sample injection temperature of 25°C at the same flow rate. In fact, the highest Mw value of MUF-A resins also appeared when the sample injection temperature was 25°C. This result is similar to the previous result in UF resins (Jeong and Park, 2017). When the sample injection temperature is 50°C, an increase in the sample injection temperature has a wide MWD as the solubility of the resin molecules increases. Therefore, the diffusion coefficient of the polymer in the solution increases as the dispersion molecules in the elution solvent increases.
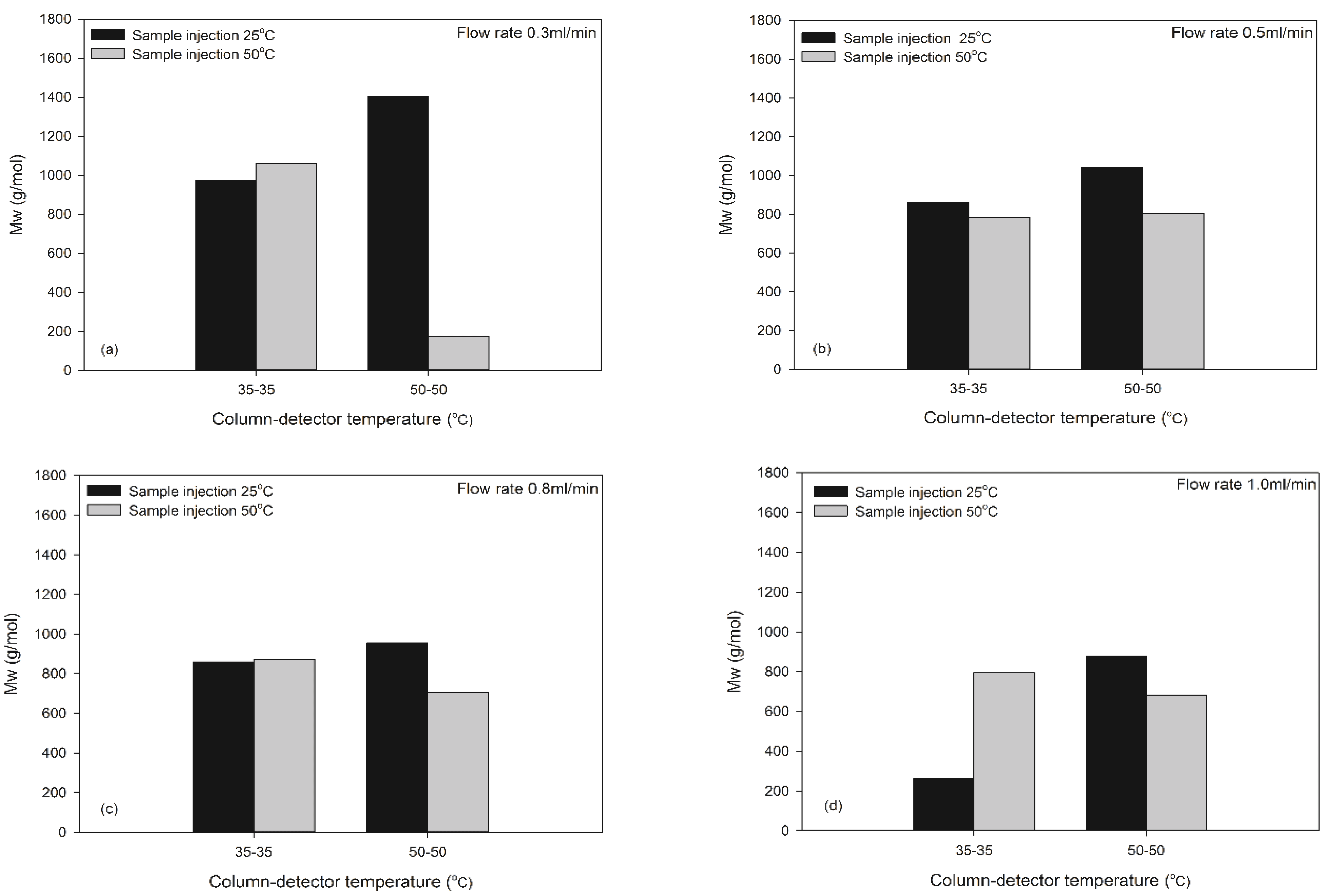
Fig. 6 is the Mw of MUF-B resins with 10% melamine at different sample injection temperatures. The MUF-B resins also have greater Mw values when the sample injection temperature is 25°C. In addition, the Mw also increases when the sample injection temperature increases to 50°C at the same C-D temperature of 50- 50°C. It can be inferred that the MUF-B resins are less branched and the resins are more soluble and dissolved in the GPC mobile phase, allowing a higher Mw.
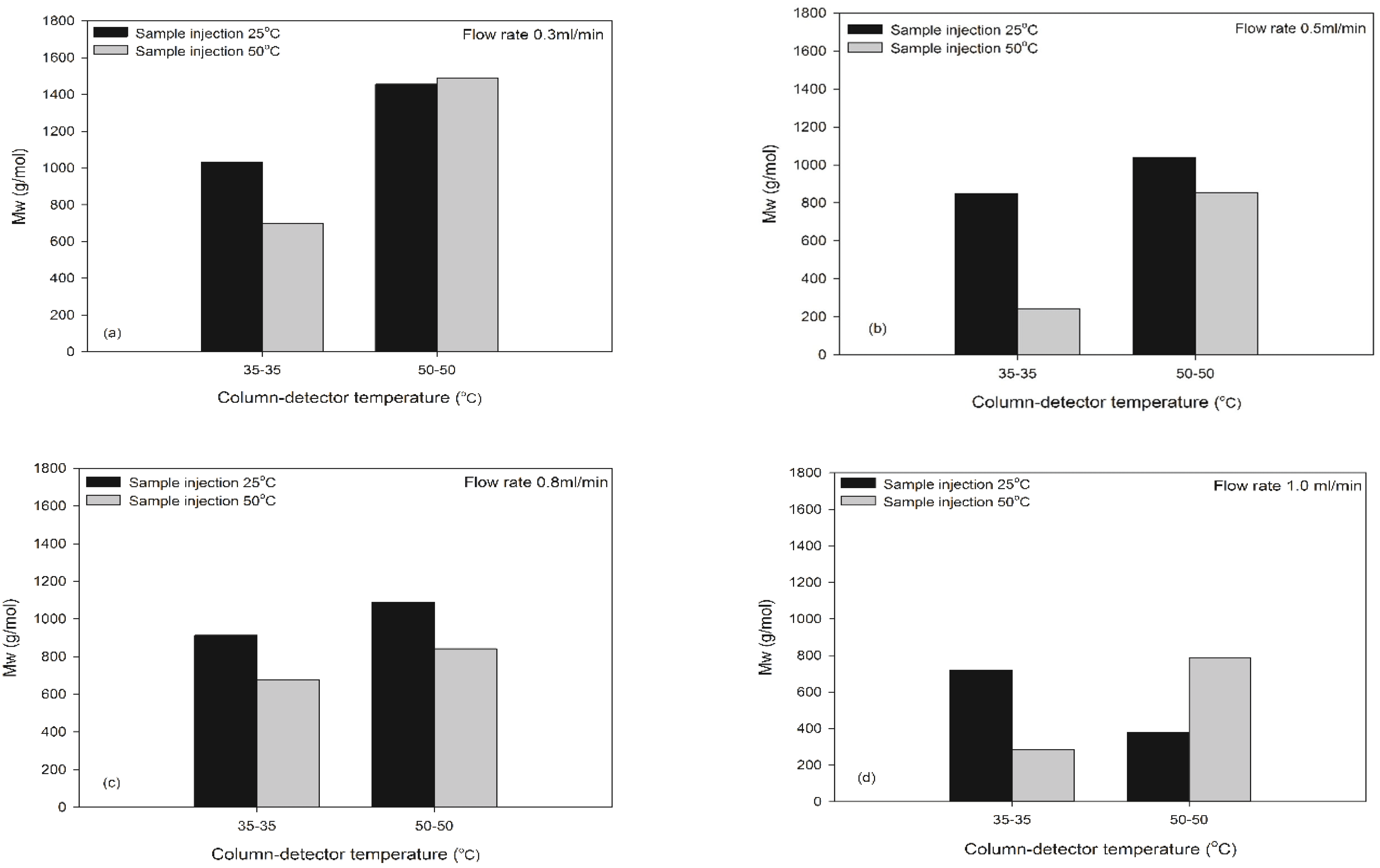
As mentioned, two different methods of synthesizing MUF reisns showed quite different distirbutions of molar mass and molecular weights, which was illustrated in Fig. 7. MUF-A resins are believed to be more or less linear molecular structure because urea reacts with formaldehyde more easily and faster than melamine does. By contrast, MUF-B resins are considered to have more branched molecular structrue than that of MUF-A resins because melamine, which has a relatively slow reaction, is reacted with formaldehyde, and then urea after a sufficient reaction time. Therefore, it is inferred that the MUF-B resins have high Mn and Mw than the MUF-A resins.
4. CONCLUSION
In this study, the effects of GPC parameters, melamine content, and resin synthesis method on the MW of the MUF resins was investigated. Both MUF-A and MUF-B resins showed the highest molecular weight when the flow rate was low. The MUF-A resins synthesized by the simultaneous reaction showed the highest molecular weight when the melamine content was 10%, but the MUF-B resins synthesized by the multi-step reaction had the highest Mw when the melamine content was 20%. However, both resins had the largest PDI when the melamine content was 10%. Meanwhile, it was found that the GPC parameters greatly influenced the molecular weight measurement results, regardless of the synthesis method. The MW was higher at the column-detector temperature was higher at 50-50°C than at 35-35°C. However, when the sample injection temperature of MUF-A was 25°C, the molecular weight was usually higher. In contrast, MUF-B resins had a higher molecular weight when the sample injection temperature was 50°C. The lower the flow rate of the MUF-A resins, the higher the molecular weight was measured when the melamine content increased to 10%. Meanwhile, the higher the molecular weight of the MUF-B resins was detected at lower the flow rate, the highest melamine content, and higher the column-detector temperature and sample injection temperature. These results showed an optimum GPC condition of analyzing the MW of MUF resins as 0.5 ml/min of flow rate, 50-50°C of column-detector temperature and 50°C of sample injection temperature, which provided a consistent MW measurement.