1. INTRODUCTION
The charcoal fuel for igniting commonly called ‘beongaetan’ in Korea is defined as ‘molded charcoal for kindling holded-coal-briquette’ which is molded to the holed coal briquette by combining bonding agent or coupling agent to sawdust charcoal in the Korea Forest Research Institute’s notification ‘Specification and Quality Standard of Wood Products’(no. 2020-1) [annex 14]. Materials such as charcoal or cokes, etc. are representative materials for facilitating surface combustion without flame, where incomplete combustion reaction occurs and carbon monoxide is producedas oxygen passes through a solid surface (Evans and Emmons, 1977). Since carbon monoxide is a colorless, tasteless and scentless gas, its combination power with hemoglobin in blood is about 200~250 times stronger than that of oxygen, it has a risk of degrading oxygen transporting capability of blood, causing suffocation (Armin et al., 1998; Weaver, 2009). Thus, if the molded charcoal is used in an indoor environment with limited oxygen supply for household heating or cooking, the users might show delayed neuropsychiatric symptoms or die due to carbon monoxide addiction (Hampson et al., 1994; Kim et al., 2015; Kim et al., 2016). Furthermore, a recognition is formed that if the molded charcoal including so called ‘beongaetan’ that generates carbon monoxide is used for suicide, people could die without any pain, and suicide cases using the molded charcoal often occur in Asian countries such as Hong Kong, Taiwan, and Japan, etc. In case of Korea, it was known that the number of suicide cases using the molded charcoal is second to the cases of using drug, agricultural pesticides, and knives, etc. (Sung et al., 2015). In order to prevent suicides using the molded charcoal, measures for restricting its sales approach are being prepared, and a product property improvement study for reducing the carbon monoxide that occurs during the combustion process is being conducted (Lee et al., 2016; Kang et al., 2018).
Methods which could reduce the amount of carbon monoxide generated include a method of adding combustion promoting substance that facilitates a complete combustion of the charcoal as the main raw material, or a method of using an alternative combustion material besides the for a complete combustion.
Torrefied wood is an intermediate phase material between wood and charcoal, and refers to a material obtained after a relatively lower heat-treatment process of about 200~300°C in an anaerobic external condition (Bourgois and Guyonnet, 1988; Pourmaekhdomi, 2014). Because this torrefied wood has a relatively higher energy density than the wood at an initial state, and hydrophobic like charcoal, it is evaluated as an excellent fuel material. It is also being utilized as a briquette for cooking barbeque (Pach et al., 2002). Apart from this, its flexibility for various uses such as antioxidant, blended coagulants for water treatment, etc. is being mentioned, and it is being used as a study material for various perspectives (Nam et al., 2018; Yang et al., 2019; Park and Kang, 2020). As such, there is a proof that the torrefied wood has an excellent fuel characteristic along with the charcoal, and higher oxygen content and volatile matter than the charcoal theoretically. Thus, it could facilitate a relatively normal combustion to reduce the generation of carbon monoxide. It is necessary to explore a suitable alternative excluding starch, etc. which is used as the existing bonding agent.
Therefore, this study designated the main component of the molded charcoal as an alternative torrefied wood, and evaluated its feasibility as an alternative material. Considering the molded charcoal products being sold in the market mainly used broad-leaved species, the Quercus was selected, and torrefied to produce the main component. Moreover, evaluations were performed on its fuel performance when it was used in combination of natural combustion improver, as well as the amount of carbon monoxide generated among the harmful gases which emerge during the combustion process.
2. MATERIALS and METHODS
The raw material used for torrefaction is a wood chip of quercus serrata (Thunb. Ex Murray) which is a hardwood species supplied from Namwon Forest Association located in Namwon, Jeollanam-do. The wood chips were dried at temperature of 105 ± 3°C for over 48 hours. For torrefaction, a wood roaster which was built independently for this research was used, and the schematic diagram is shown in the Fig. 1. The circular frame of the system indicated in the schematic diagram is a reactor, and a rotary drive was rotating at the speed of 45 rpm through a rotation axis located in the center of the reactor while oxygen from outside was blocked using a hydraulic cylinder. Then, the reactor was heated through a direct heat generated from a heat supply apparatus located at the bottom of the reactor. Once the internal temperature reached 220°C, the wood chips were inserted by opening the upper hatch. The torrefaction time was set as 300 seconds each, and the entire torrefied wood chips were pulverized using a mixer. The pulverized matter passed through #8 standard screen, and it was dried for over 48 hours at 105 ± 3°C once again.
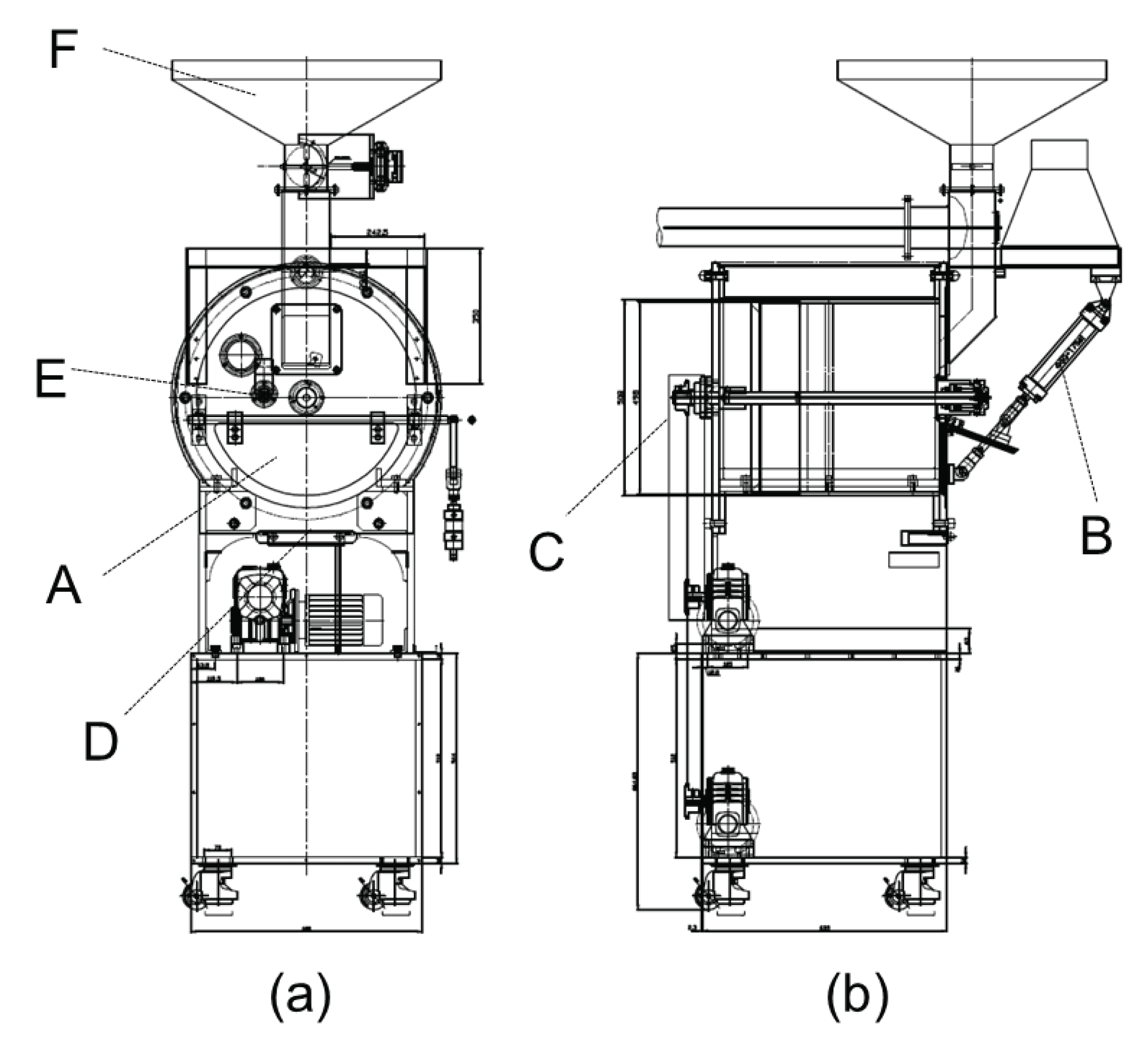
For the material used as an adhesive for specimen production, gelatin solids extracted from protein of a pig (Deokyoung Gelatin, Pocheon in Gyeonggi-do) was purchased from a market and used. This is made up of 84~90% of protein, 8~12% of water, and 2~4% of mineral. Distilled water was combined with the gelatin solids to produce it as a liquid form, and mixed amount of the distilled water was set as twice the number of solids of gelatin. Then, the mixture was stored in a container, sufficiently stirred until the gelatin solids are completely dissolved within a constant temperature water bath with the condition of 45 ± 3°C, and used as the adhesive of specimen.
For forming and injection processes of the specimen, the self-produced hydraulic press was used. The target density of the specimen was 0.7 g/cm3, and considering that the internal volume of 13-pin mold frame was 427.21 cm3, the gelatin solids content was set as 15 wt% in a torrefied wood dry weight of 300 g. At this point, since the moisture content of gelatin solids was 12%, the mixture was produced by considering moisture correction value. Then, the mixture was inserted in the 13-pin mold frame, pressure of 0.78 MPa was applied. The gelatin adhesive was cold pressured for 600 seconds so that it could be hardened sufficiently at room temperature. These were collected, and cured for 168 hours at room temperature.
For the combustion promoting substance of the specimen, flaxseed oil, a dry fat oil contained in the seeds of flax, was purchased from the market and used (Daemyeong Chemicals Co., Ltd. Seoul). This is made up of 8~9% of saturated acid, 10~15% of oleic acid, 25~35% of linoleic acid, 35~45% of linolenic acid, 6.5% of oxygen, 0.5~1.5% of non-unsaponifiable matter, and 4.5% of glycerol. 20 g of flaxseed oil was applied on a single surface of the specimen after the room-temperature dry treatment was completed. Then, all specimens were dried for over 24 hours at 105 ± 3°C. In addition, as a control group of the specimen, molded charcoal (“H” company, Korea) purchased from the market was selected. The control group specimen was configured with charcoal powder, barium nitrate, potassium nitrate, and whole meal. The properties (mass, moisture content percentage, and density) and description of each specimen were indicated in the Table 1 and Fig. 2.
Specimen | Mass (g) | Moisture content (%) | Density (g/cm3) |
---|---|---|---|
HTWB1) | 319 ± 2 | 16.0 ± 0.5 | 0.69 ± 0.01 |
MC2) | 340 ± 3 | 1.7 ± 0.3 | 0.65 ± 0.01 |
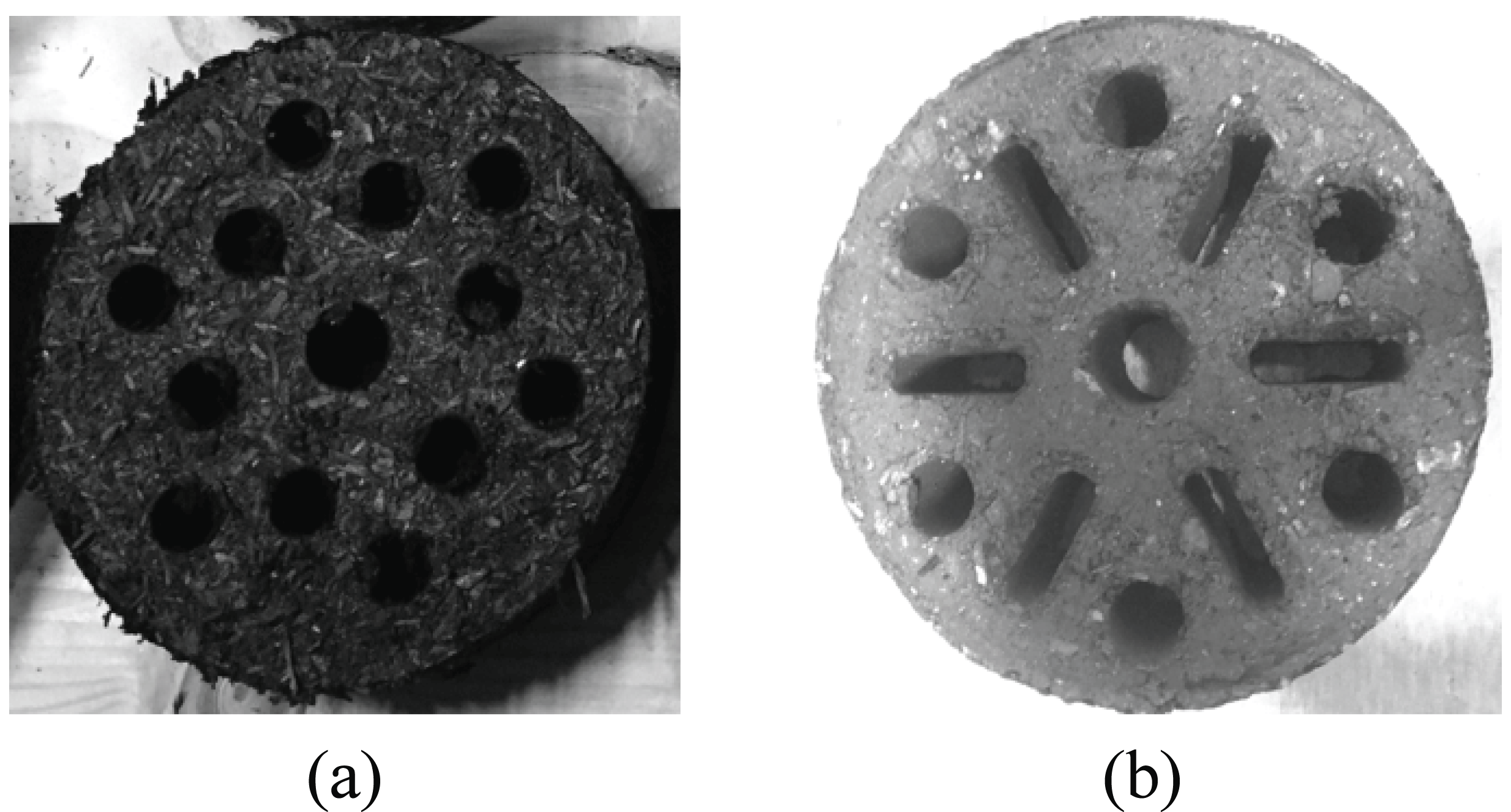
The elemantal analysis was conducted according to test standard ASTM D3176-74, and the test was performed through an elemental analyzer EA 1108 CHNS-O (FISON Instruments, USA). For the test condition, the powder specimen was instantaneously combusted in a pure oxygen atmosphere for oxidation, the generated gas compounds were separated by their components from a separation tube of the equipment, and a quantitative analysis was performed on C, H, N, S among the specimen through TCD (thermal conductivity detector). At this point, O was generated by pyrolysis, transformed into carbon monoxide, and redetected by TCD again. The measurement was performed 3 times repeatedly, and the average value of each item was estimated.
The ash content measurement was conducted by test standard ASTM D3174-04, and the test was performed through Muffle Furnace (CEBER CERAMIC FIB, USA). For the test method and condition, a crucible containing the specimen was inserted into the muffle furnace, and the internal temperature of the furnace was increased up to 250°C at speed of 4~5°C/min, and the crucible was left for 1 hour. Then, the temperature was increased (at approximately 9~10°C/min) to reach up to 800 ± 25°C for 1 hour, and maintained that temperature for minimum of 2 hours. The crucible was removed from the furnace, left in the air for 300~600 seconds, cooled down to room temperature, and its weight was measured at 0.1 mg level and recorded. The measurement was performed 3 times repeatedly, and the average value of the measurements was estimated.
HHV (higher heating value) measurement was performed according to test standard ASTM D5865/ D5865M-19, and the test was performed through a cylinder-type calorimeter Parr 6400 (PARR INSTRUMENT INC., USA). At this point, the specimens which were pulverized by the mixer and passed through #16 standard screen were used for both conditions, and temperature increase rate during the combustion process of the specimens by the cylinder-type calorimeter was measured to estimate HHV (MJ/kg). The measurement was performed 3 times repeatedly, and the average value of the measurements was estimated and indicated.
In order to detect harmful gases content that emerge during the combustion process of each specimen, a combustion gases measurement test was performed as shown in the Fig. 3 below. After the specimen of each condition which was chelated in outdoor environment was fixed within 1,000 × 1,300 × 2,000 mm chamber, an air emission measuring instrument GREENLINE MK2 (EUROTRON, England) was used to capture the combustion gases of the specimen through an injection tube of the instrument, and measure the gases. Three gases were assessed, including carbon monoxide (CO), nitrogen oxide (NOx), and sulfur dioxide (SO2). At this point, nitrogen dioxide (NO2) was derived from measuring each carbon monoxide (NO) and nitrogen dioxide (NO2) for 900 seconds. In order to perform a simulated experiment of conditions in which the molded charcoal distributed in the market was misused for suicide, this study carried in the ignited specimen in the chamber with oxygen concentration of 20.8%, and sealed the chamber to perform the combustion gases emission measurement.
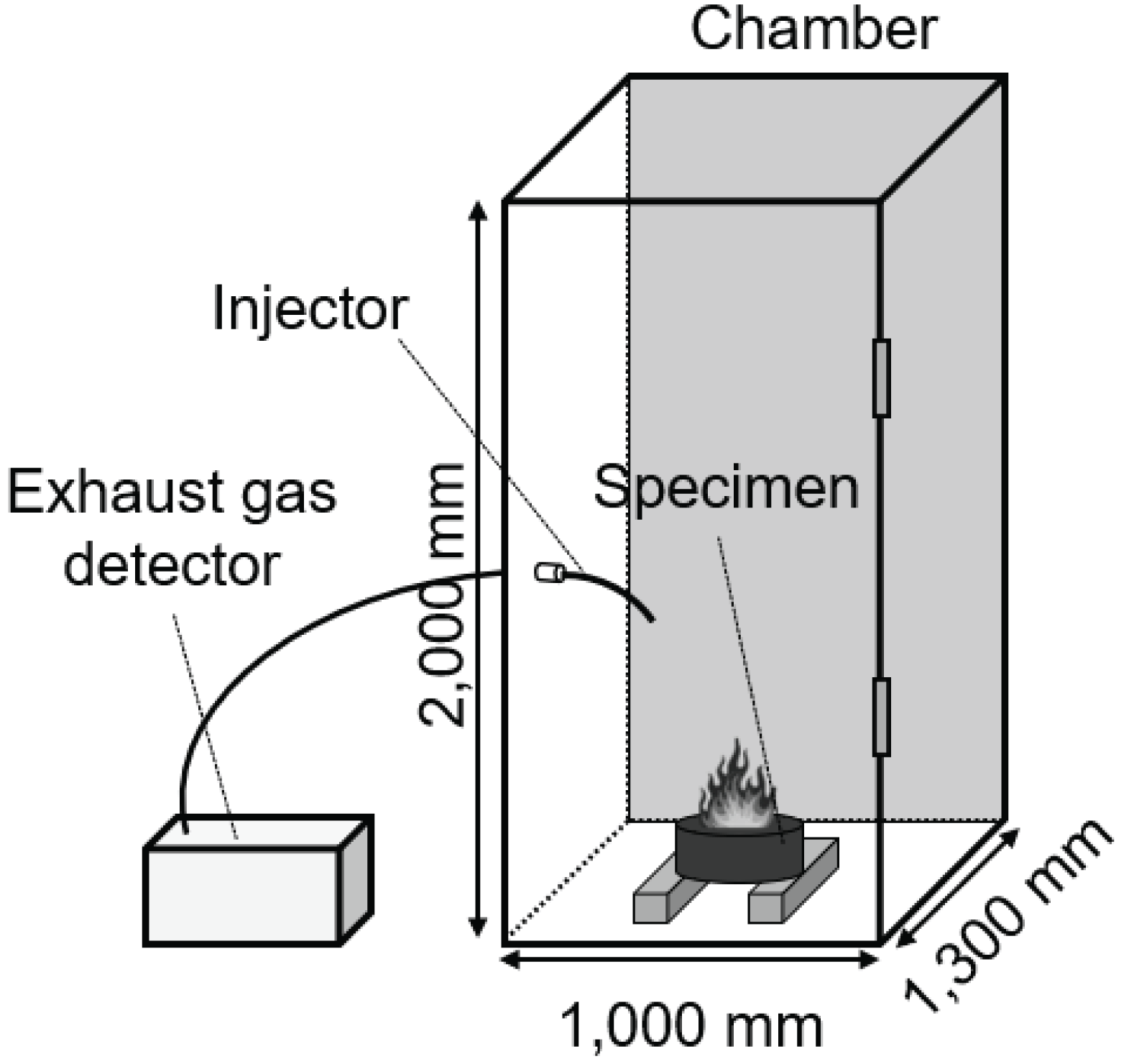
3. RESULTS and DISCUSSION
Table 2 indicates the analysis results of elementary analysis, ash content and higher heating value. These measurement results were obtained in a condition where the torrefied specimen included the gelatin and flaxseed oil, and the molded charcoal available in the market included barium nitrate and potassium nitrate. Sulfur was not detected from the specimen in both conditions, and the carbon content was about 50%, which indicated no big difference between the conditions. In contrast, for hydrogen and oxygen contents, it was revealed that the torrefied specimen had higher contents than the molded charcoal by approximately 2.6 and 2.2 times, respectively. On the other hand, the torrefied specimen had a lower nitrogen content than the molded charcoal distributed in the market by approximately 2.3 times.
The result of ash content indicated that the torrefied specimen had a lower content than the molded charcoal distributed in the market by about 51 times. It is determined that this result was indicated because the molded charcoal which is being distributed in the market has a relatively higher non-volatile matter, considering the fact that it includes approximately 25 wt% of the metal structural materials barium nitrate, and also the combustion promoting substance potassium nitrate and the adhesive whole meal, etc. along with hardwood charcoal.
The result of higher heating value indicated that the torrefied specimen had a higher value than the molded charcoal distributed in the market by about 14%. According to a precedent study by Boley and Landers (1969), hardwood charcoal produced at temperature range of 571~650°C had carbon content of approximately 65~68%, higher heating value of 23~25 MJ/kg, and ash content of 15~17%. Comparing these results with the molded charcoal in the market used in this study, despite its main component was hardwood charcoal, the carbon content and higher heating value were lower by 26~29% and 25~31%, respectively, and ash content was higher by 30~39%. These results are also considered to be due to the previously mentioned fact that molded charcoal includes metal structural materials of barium nitrate and other additives, thus it has less quantity of elements which could contribute to heating of hardwood charcoal, the main component. However, the ignition time of hardwood charcoal is more delayed than that of torrefied wood by approximately 50%, and the hardwood is unable to facilitate flaming combustion independently (Lee, 2016).
Considering the fact that the molded charcoal shall add metal structural materials such as barium nitrate for the purpose of the product, it is determined that fuel performances of the torrefied specimen formed by using gelatin produced in this study and the combustion promoting substance flaxseed oil are more excellent.
For the combustion phase of each specimen, the molded charcoal in the market caused flame combustion with combustion promoting substance such as barium nitrate, etc. at the time of ignition, and the combustion phase was changed to surface combustion, which stayed until the last session of the test. For the torrefied specimen on the other hand, it caused flame combustion until the last session of the test even after the ignition, and the combustion flame was close to dark red to red.
Fig. 4 shows the result of indicating the amount of carbon monoxide generated during the combustion process of each specimen by time unit. It was revealed that the amount of carbon monoxide generated from the molded charcoal in the market was increasing remarkably since the beginning of the combustion process, and this is determined to be caused by incomplete combustion reaction by surface combustion. In the qualitative analysis test of combustion gases, the maximum amount of carbon monoxide was 718 ppm and 4,096 ppm in the last 900 seconds session for torrefied specimen and molded charcoal in the market, respectively. The torrefied specimen showed approximately 50 times reduced effect compared to molded charcoal in the market. Tumuluru et al. (2010) reported that biomass of the same species has volatile matter content of carbide reduced as it has a high heat-treatment temperature, and a longer reaction time. In addition, the result of a study by Lee (2016) identified that a torrefied specimen of oak which includes gelatin and flaxseed oil has approximately 48% greater amount of carbide than the molded charcoal.
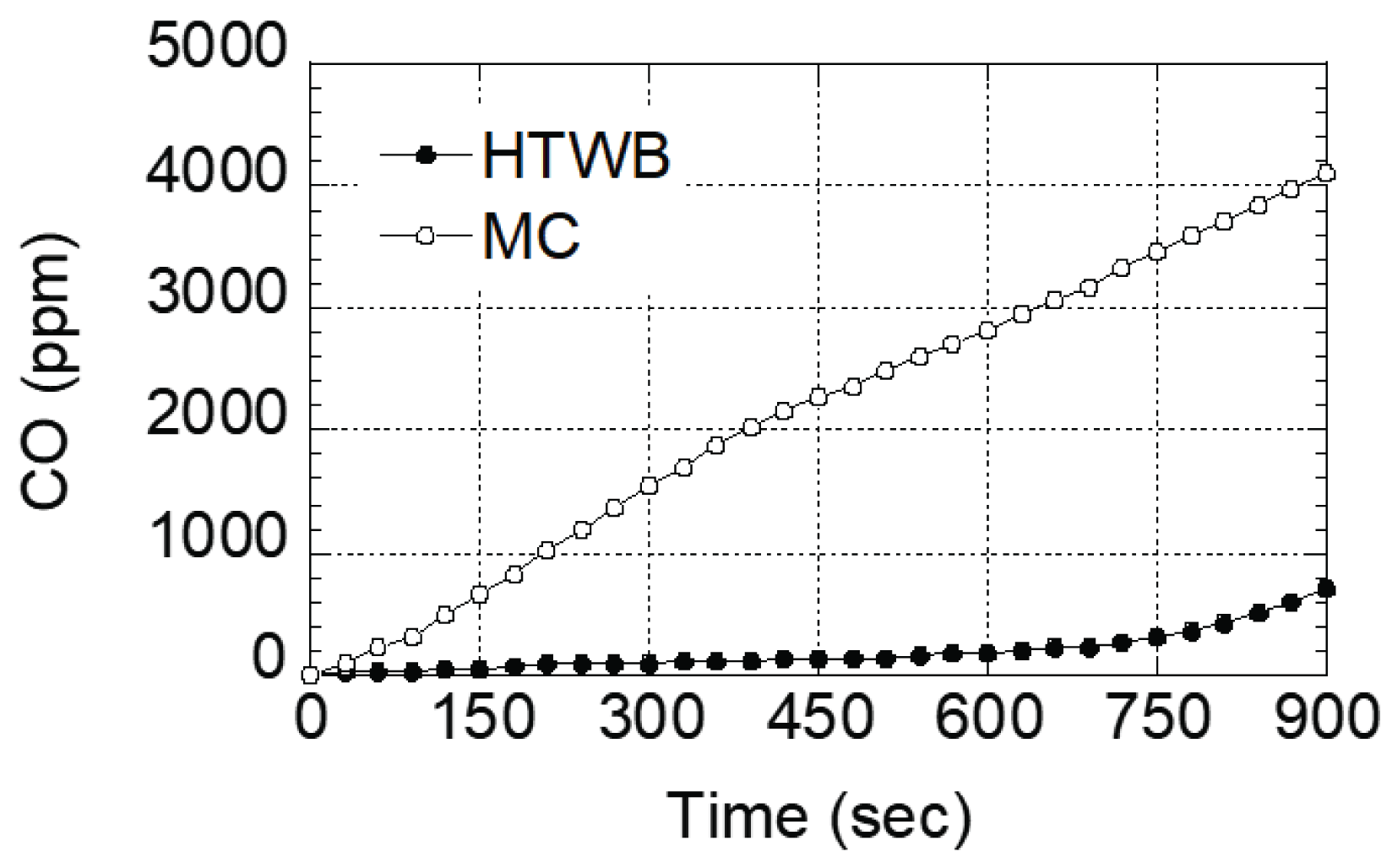
Considering each of these results, since the contents of the torrefied specimen contain a relatively larger amount of carbide than the molded charcoal in the market, it shows a relatively complete combustion reaction, and thus carbon monoxide content was remarkably lower.
Furthermore, oxygen concentration in the chamber during the combustion process of each specimen was shown in the Fig. 5. Both specimens showed reduced oxygen concentration with the delayed combustion time. However, oxygen concentration of molded charcoal reduced gradually in a linear form until the end of the combustion test, while that of torrefied specimen reduced relatively rapidly in a curved form until approximately at 500 second, when the concentration was almost maintained.
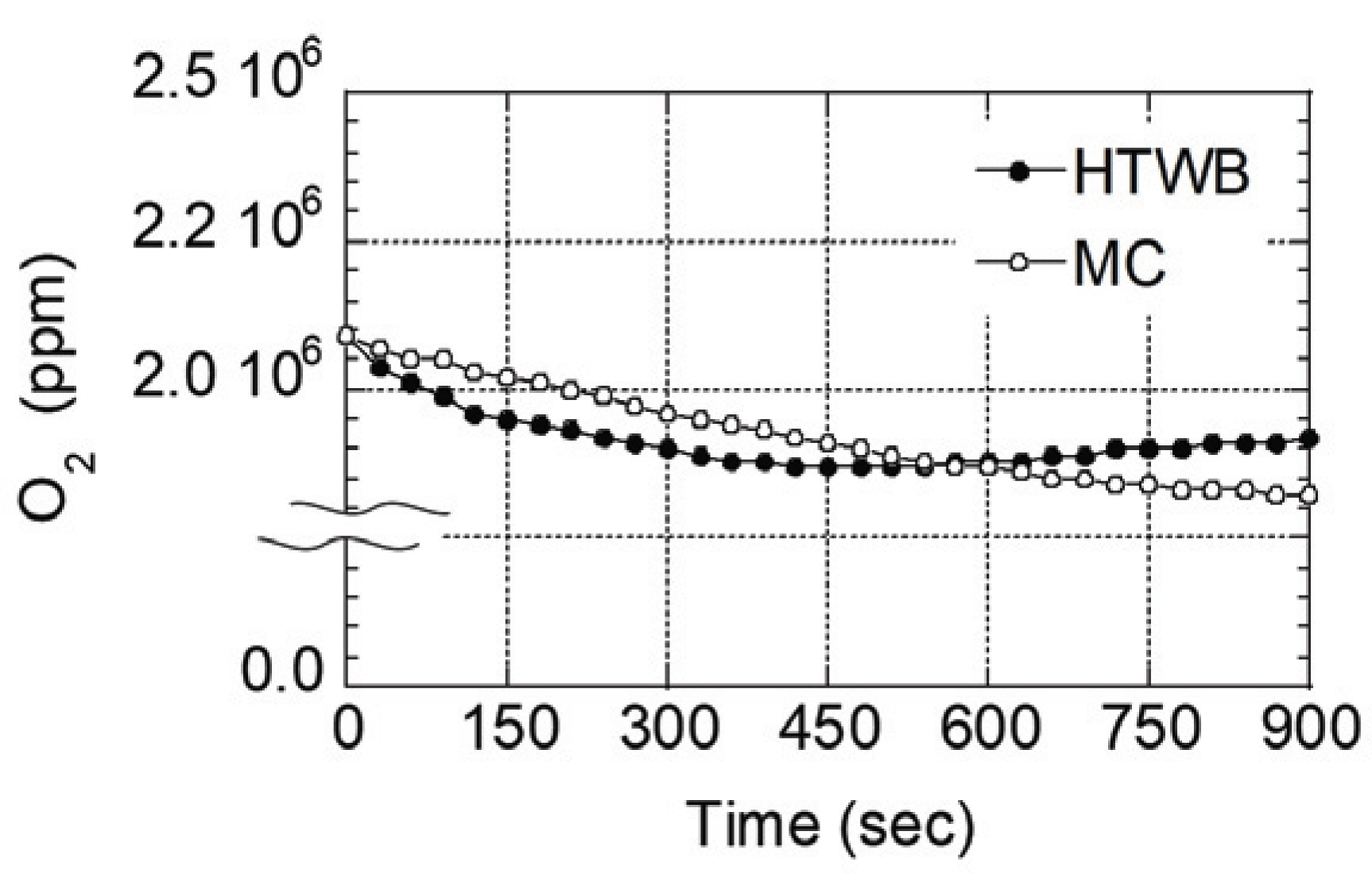
Fig. 6 is the result of indicating the amount of nitrogen oxide and sulfur dioxide generated during the combustion process of each specimen by time unit. It was found that nitrogen oxide of torrefied specimen increased slowly as it drew a curved from since the beginning of combustion, and the amount was approximately 2 times larger than that of molded charcoal distributed in the market since it passed 200 seconds. In contrary, nitrogen oxide of molded charcoal distributed in the market was revealed to indicate a lineal form since it passed 100 seconds, and the value was maintained after. The cause for this result is due to combustion phase (flame combustion and surface combustion) of each specimen. In addition, considering the fact that flame of the previously mentioned torrefied specimen was close to dark red to red, the flame temperature is determined to be approximately at 525~1,000°C (Stirling, 1905). Gadi et al. (2003) reported that the cause of high amount of NOx generated during the combustion process of biomass is due to flame combustion, and NO’s thermoforming occurs due to reactions of atmospheric nitrogen and oxygen at high temperature. As such, it was determined that torrefied specimen having flame combustion phase had a relatively high amount of NO generated than molded charcoal distributed in the market, which has surface combustion phase. Moreover, it was revealed that the amount of sulfur dioxide generated was quite small or almost none for both specimens.
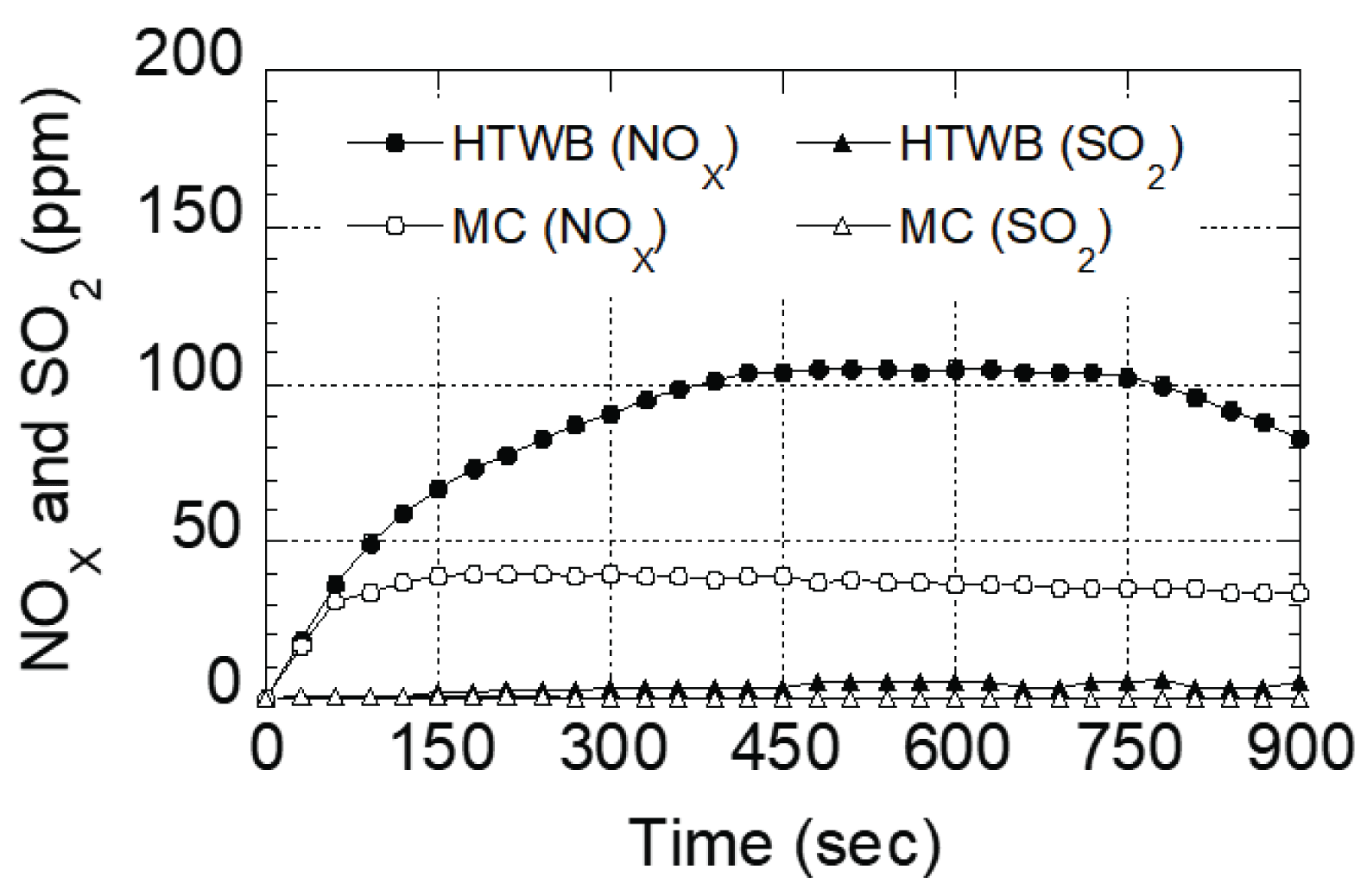
Next, the relationship between the carbon monoxide concentration and the human health hazard identifiedfrom studies by Struttmann et al. (1998) and Goldstein (2008) was cited to compare the amount of carbon monoxide generated in the two specimens of this study. These studies revealed that if the concentration of carbon monoxide is 3,200 ppm, people will show symptoms of headache, dizziness, and nausea within 5 to 10 minutes, and lead to death within 30 minutes if they are continuously exposed to such environment. As such, considering that the maximum value of the amount of carbon monoxide generated in the molded charcoal distributed in the market was 4,096 ppm, it means that people are highly likely to die within 30 minutes. On the other hand, the maximum value of the amount of carbon monoxide generated in the torrefied specimen was 718 ppm. According to a precedent study in the similar range, people show symptoms of dizziness, nausea, and convulsion within 45 minutes at 800 ppm, and lose bodily sensation within 2 hours, but still alive. The torrefied specimen manufactured as an alternative material of molded charcoal using torrefied wood (an intermediate material between wood and charcoal), protein-based gelatin adhesive and flaxseed oil (dry fatty oil) in this study has a far lesser amount of carbon monoxide generated during the combustion process than molded charcoal for roasting which is distributed in the market. Therefore, it is expected that even if it is misused for suicide, its success rate will be largely reduced.
4. CONCLUSION
This study used gelatin adhesive to cold press a wood chip of a torrefied oak for 300 seconds at 220°C, and applied flaxseed oil to produce a specimen. The results of fuel characteristics and a quantitative assessment of combustion gases for comparing performances between the specimen and molded charcoal which is available in the market are as follows:
The carbon content of torrefied specimen was approximately 50%, which had no big difference with that of molded charcoal in the market, and it was confirmed that the hydrogen and oxygen contents were 2.6 times and 2.2 times greater, respectively. The nitrogen content of torrefied specimen was about 2.3 times lesser than that of molded charcoal in the market.
It was revealed that the ash content of torrefied specimen was about 51 times lesser than that of molded charcoal in the market, and the higher heating value of torrefied specimen was confirmed to be about 14% larger.
Since the maximum value of the amount of carbon monoxide generated in the specimen through the combustion process for 900 seconds was about 50 times lower than that of molded charcoal in the market, it was confirmed that it showed a quite large carbon monoxide reduction effect.
Considering the fact that people only experience sensory paralysis and no death even if they are exposed to the torrefied specimen of this study for 2 hours, it implies that even if this is misused for suicide, it could reduce the success rate.