1. INTRODUCTION
Recently, with the improvement of social welfare, interest in noise in the living environment is increasing. One of the main requirements for a comfortable human life in a complex and modern society is sound absorption. The demand for the development of ecofriendly sound absorbing materials is gradually increasing for high sound proof driving in automobiles, manufacturing environments, and equipment (Ersoy and Küçük, 2009; Bujoreanu et al., 2017; Koizumi et al., 2002). Industrial sound absorption generally uses these materials, such as sponge, glass or rock wool, foam, fabric, and synthetic fibers. In case of sound absorbers made of glass fiber and rock, micro-particles caused by weathering may cause air pollution during exposure to the air, and there are disadvantages of difficult reuse and incineration treatment during disposal.
In addition, the bond strength between fibers may be weak, causing damage to transportation and handling, and direct contact with the human body due to sweat or moisture may cause irritation or itching and, in severe cases, skin cancer (Lee. 2001).
Therefore, for the health of human beings and the environment, the development of natural, cheap, renewable and abundant alternative materials is needed, and the whole world is paying attention to the development of eco-friendly materials. As the related research progresses, interest in abundant forest resources is naturally increasing, and the demand for research on improving the noise absorption performance of wood and wood products as an eco-friendly material is gradually increasing(Byeon et al., 2010). Improvement of sound absorption capability of wood and wood- based board by resonant absorption was attempted, and stair type perforation higher than a normal specimen (Kang and Park, 2001). Betula costata Trautv and other species additional experiments were found to closely correlate the size, shape, and arrangement of resonators, the structural direction and gravity of wood, and the sound absorption frequency domain (Hang et al., 2008). Carbonization treatment was performed to improve sound absorption property for MDF at carbonizing temperatures of 500°C ~ 1,100°C. and the sound absorption coefficient increased between the temperatures of 500°C and 900°C, but decreased at a temperature of 1,100°C. (Won et al., 2015). Using the Wise's method, the wood by delignification treatment was made small cracks, which increased the sound absorption in a specific frequency range (Kang and Lee, 2005).
Wood bark was determined that by adjusting the thickness and apparent specific density and attaching plywood at the back, wood bark particle mats generally offer excellent sound absorption and insulation performance (Kang et al., 2019). The oak mushroom bed log showed sound absorption was two to three times higher than normal oaks wood in the frequency range of 2~6 kHz (Kang et al., 2008). Bamboo fiber material was measured at the influences of thickness, air space depth, apparent density and bamboo fiber diameter using an impedance tube. Results showed that sound absorption coefficient of the bamboo fiberboard and the resonance-type bamboo fiberboard was found to be superior, especially in the high-frequency range (Koizumi et al., 2002). The noise reduction coefficient of rice hull mats was approximately 0.8, indicating a high sound-absorption property of the mats, therefore, rice straw matting is a suitable candidate material for sound-barrier walls against highway noise (Kang et al., 2019). The sound absorption coefficient of flame resistant treated sawdust-mandarin peel composite particleboard was lower than that of untreated specimen, but higher than that of gypsum board (Kang and Kang, 2015). Coconut coir fiber compressed into bales and mattress sheet was found to demonstrate good sound absorption coefficient. When compared to a single layer, multi-layer coconut coir fibers with airspace layers increase the absorption coefficient of the material at lower frequencies (Nor et al., 2004).
In addition, as a result of analyzing the noise reduction effect of trees in the surrounding living environment (Kim and Oh, 1994), roadside forests such as mixed forests and willow shrubs have been reported to attenuate noise of more than 15dB.
The main objective of the present study is to compare and analyze the sound absorption effect according to the leaf size and layer thickness of evergreen broadleaf trees as a natural sound insulation material.
2. MATERIALS and METHODS
To investigate the sound-absorbing properties of the evergreen hardwood leaves depending on the size and thickness of specimens, the leaves of Korean dendropanax (Dendropanax morbiferus) and Japanese aralia (Fatsia japonica) were collected in National Institute of Forest Science (Jinju). Two evergreen broad leaved trees of D. morbiferus and F. japonica are mostly distributed in subtropical and warm temperate forest zone in Korea. Before this experiment, three samples for species were collected and estimated using thickness measuring instrument (PDN-21, Peacock, Japan) and leaf area measuring instrument (Li-3000C, Licor). The basic data are shown in Table 1, the mean leaf area of D. morbiferus 1370.36 cm2 was greater than that of F. japonica 14.83 cm2, F. japonica 1.279 mm and D. morbiferus 0.632 mm in mean leaf thickness. The mean moisture content was higher 243.35% in D. morbiferus leaves than 207.28% in F. japonica leaves. It is believed that these leaf characteristics are due to genetic growth characteristics between these two species.
Classification | Leaf area | Length | Width | Thickness | Moisture content |
---|---|---|---|---|---|
(cm2) | (cm) | (cm) | (mm) | (%) | |
D. morbiferus | 60.03 | 11.30 | 5.03 | 0.632 | 243.35 |
F. japonica | 1370.36 | 138.83 | 18.20 | 1.279 | 207.28 |
The specimen was cut into three types, 0.5 × 0.5 cm2, 1.0 × 1.0 cm2, and 2.0 × 2.0 cm2 as specimen unit size, using a straw cutter for measurement. The cut specimens were sealed to maintain the initial moisture content. As shown in Fig. 1, when measuring the sound absorption, all the specimens as configurations of biomass materials (Oldham et al., 2011), were made with the same pressure (0.0256 kgf/cm2), and the case used was chosen to have very little sound absorption, the diameter of the case is 6.25 (∅) cm, the height is 5 cm.
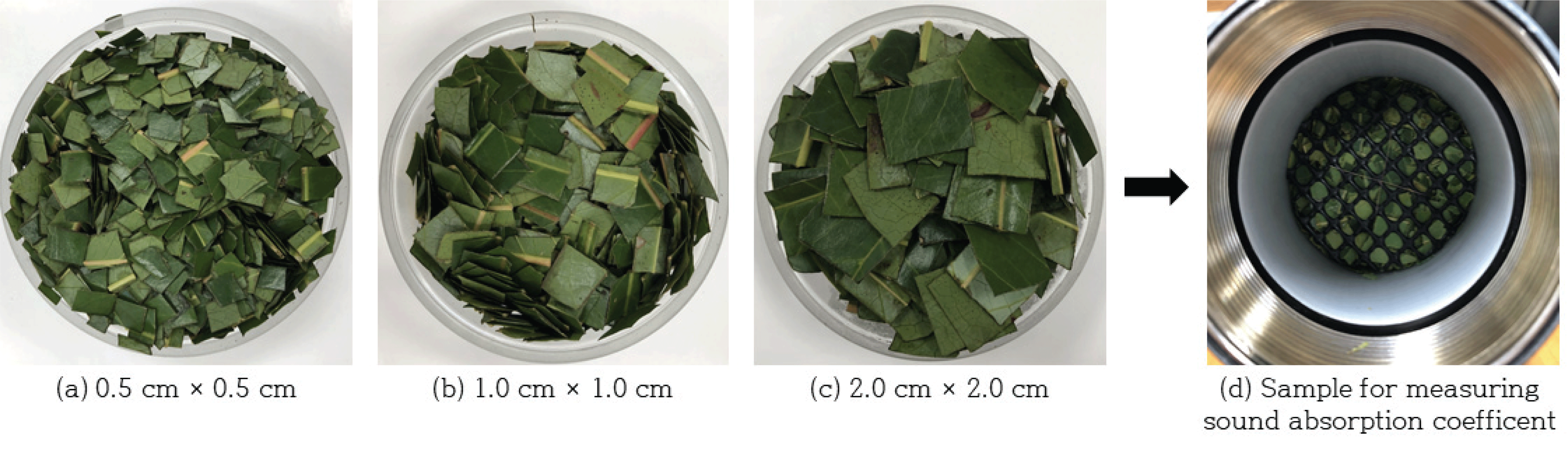
The sound absorption properties test apparatus for the two microphone method consisted of an impedance measurement tube (Type 4206A, B&K, Denmark) in the right side of Fig. 2, a sign analyzer unit (Type 2035, B&K, Denmark), a power amplifier (Type 2706, B&K, Denmark) and two microphones (Type 2670, B&K, Denmark), as shown in the left side of Fig. 2 (User manual, 2002). The range of the frequency measurement was 100 Hz~3,200 Hz in a middle type impedance measurement tube. The SAC was determined according to ASTM C 384-04 (2011).
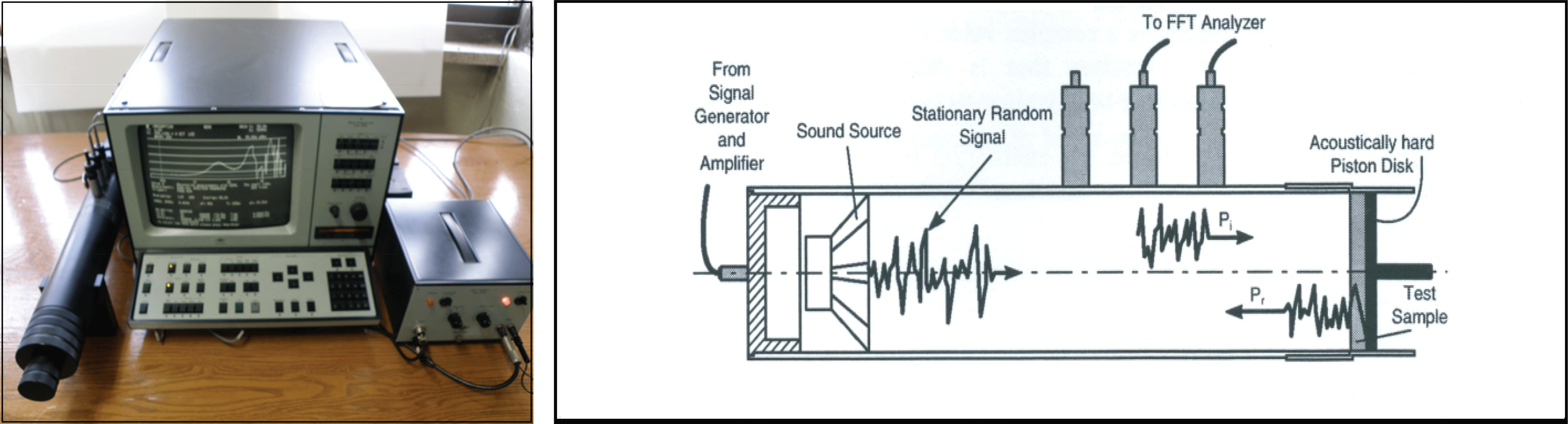
Following calibration with a sponge, the SAC of the material was measured 100 times each specimen and the mean value of the test results was estimated. Three replication tests were conducted on each condition. After the measurement of sound absorption coefficient (SAC), an average sound absorption coefficient was calculated by dividing denominator into numerator in the frequency range of the measurement using the following equation (1) (Won et al., 2015).
SACs are shown in Table 2, and the values were statistically analyzed performed by Duncan's multiple range test using PASW Statistics 18.0 (SPSS Inc., Chicago, IL, USA).
3. RESULTS and DISCUSSION
Leaf thicknesses and specimen sizes varied with thicknesses of 1.00 cm, 1.75 cm, and 2.00 cm and/or with specimen sizes of 0.5 × 0.5 cm2, 1.0 × 1.0 cm2, and 2.0 × 2.0 cm2. Table 2 and Fig. 3 show the sound absorption properties of evergreen broad-leaved tree by leaf thickness and specimen size. In general, it is reported that the higher the density per unit volume, the higher SACs for the same material (McGrory et al., 2012). Therefore, in this study, as shown in Table 2, the SACs for each species were values obtained under almost the same apparent density conditions. That is, for D. morbiferus, the apparent density is within the range of 0.28-0.30 ρ (g/cm3), and F. japonica is limited to a narrow range within the range of 0.24-0.27 ρ (g/cm3). The apparent density was intended to be based on the same conditions with constant weight and pressure during the experiment. The apparent density was measured under constant weight and pressure conditions, so the apparent density value was constant and no standard deviation occurred (Table 2).
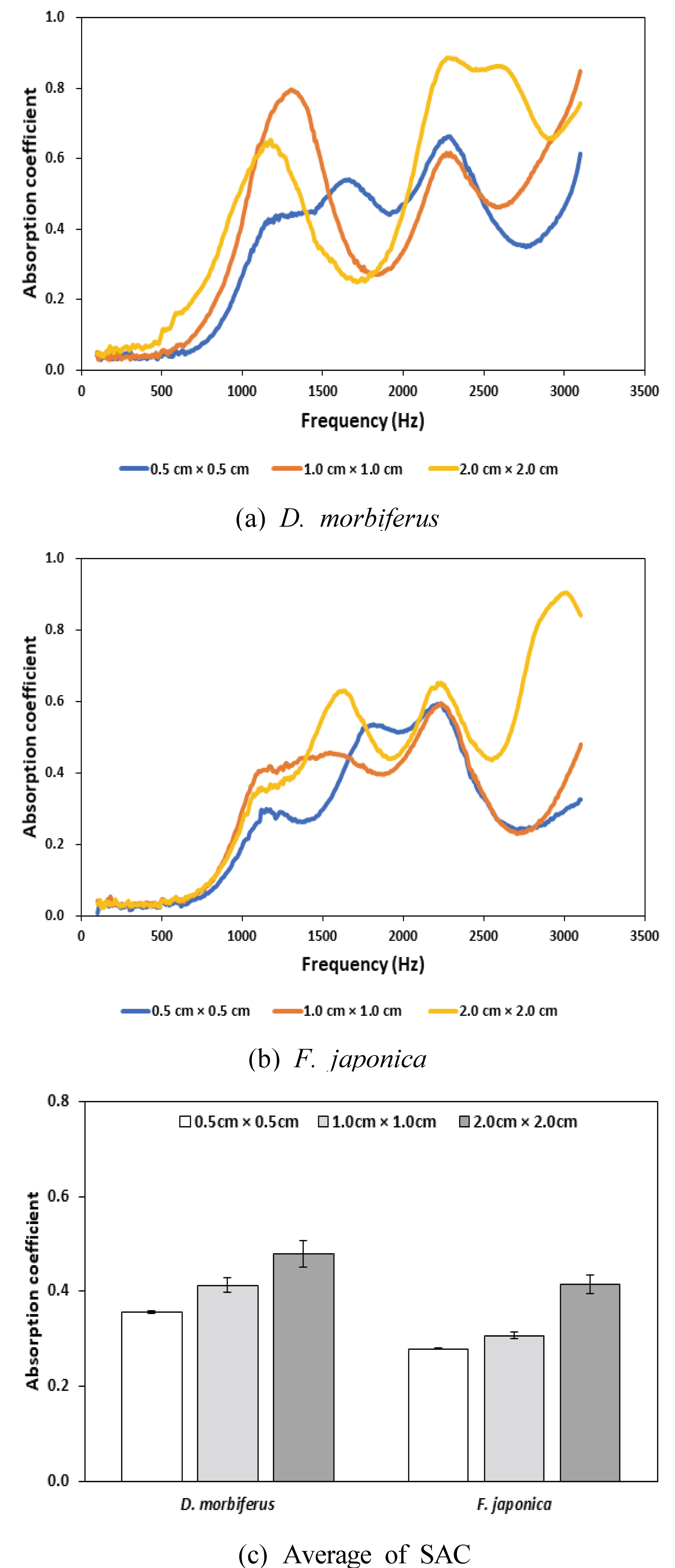
These results showed a tendency similar to the result of the increase in SAC as the sample thickness increased to 2.0 cm, 3.0 cm, and 4.0 cm in the data palm test conducted by Taban et al. (2019), and they also found that introducing an air gap behind the sample in an impedance tube can effectively improve the frequency bandwidth of absorption for thin samples.
Of all the conditions in this study, the largest mean sound absorption coefficient of 0.601 was found at 0.5 cm × 0.5 cm leaf size conditions at 2.50 cm thickness in F. japonica species. The mean SAC of F. japonica in each treatment condition ranged from 0.279 to 0.601, and 0.356 to 0.593 for D. morbiferus. Under the leaf thickness of 1.00 cm, both species showed significantly higher SACs as the leaf sample size increased (Table 2). However, as the thickness increased, the difference in sound absorption effect according to the leaf size generally tended to decrease.
In particular, in the case of D. morbiferus, there was no significant difference in the coefficient between leaf sizes under 2.5 cm thickness condition (p<0.05). In the case of F. japonica, the difference in sound absorbing effect (0.071) between 0.5 × 0.5 cm2 and 2.0 × 2.0 cm2 leaf size under the condition of 2.50 cm thickness was slightly weaker compared to the case of 1.00 cm thickness (0.135). The SAC for all conditions of D. morbiferus was higher than that of the sponge used for calibration of the experiment, 0.303. At 1.75 cm and 2.50 cm thicknesses of F. japonica, the SAC of all leaf specimen sizes was higher than that of the sponge. However, in the condition of 0.5 × 0.5 cm2 specimen size at 1.00 cm thickness of F. japonica, the SAC was exceptionally 0.279, which was lower than that of the sponge.
Fig. 3(a) ~ (b) show the difference in SAC by leaf size according to the frequency. The sound absorption graph of two species shows three peaks: 1,000 ~ 1,500 Hz, 2,000 ~ 2,500 Hz, and 3,000 Hz, respectively. It is similar to the absorption property of the sponge. SAC of 100 ~ 500 Hz is low but increases after that. These results showed a similar tendency to the results of bamboo species by Koizumi et al. (2002) that the SACs for bamboo fibers as natural porous material were high in the mid- and high-frequency ranges. The properties of these porous materials are generally reported to be good absorbers at high frequencies and poor absorbers at low frequencies (Ismail et al., 2010).
Under the condition of 1.00 cm thickness filled with a leaf size of 0.5 × 0.5 cm2, the SAC was generally low in both species, especially in the case of F. japonica, the coefficient was even lower than that of the sponge (Table 2). This occurred around 1,000 Hz, a relatively low frequency, as shown in Fig. 3. Therefore, in this study, the sound absorption effect by leaf size under 1.75 cm and 2.50 cm thickness conditions was intensively compared and analyzed for each frequency band, and the results are as follows.
The results of comparative analysis of the sound absorption effect according to the size of the leaf sample under the condition that the thickness is 1.75 cm and the variation of the SAC as the frequency increases for each species are shown in Fig. 4. In Table 2, as the leaf size of F. japonica increased, the SAC tended to increase, but the difference in value was not large. In the case of D. morbiferus, the difference in SAC by leaf size with a thickness of 1.75 cm was also weak.
At a thickness of 1.75 cm, the difference between the SACs of each leaf size was less than 0.1, but this result showed significantly higher values than the SAC of the same leaf size condition at a thickness of 1.00 cm. Average sound absorption coefficients range from 0.422 to 0.564 with a thickness of 1.75 cm of F. japonica.
Fig. 4(a) and (b) show the result of comparing the variation of the SAC with the increase in frequency for each species and sponge. In both species, the SAC increased rapidly from 500 Hz, reaching mostly 1.0 in the 2,000~2,500 Hz range. And as the frequency increased from 1,000 HZ, the difference in SAC by leaf size was larger in F. japonica than in D. morbiferus. That is, the change in SAC for each frequency according to leaf size conditions was higher in F. japonica species. The variation characteristics of the SACs according to the increase in the frequency showed a similar trend in the conditions of 1.75 cm and 2.50 cm in thickness, but showed slightly different characteristics in the 1.00 cm thickness condition. From the above results, the difference in SAC according to the increase in frequency by leaf size was more sensitive and consistent in the F. japonica than in the D. morbiferus, and the SAC tended to increase as the frequency increased in the F. japonica. It is thought that this difference characteristic comes from the difference in structural characteristics of the leaves between two species.
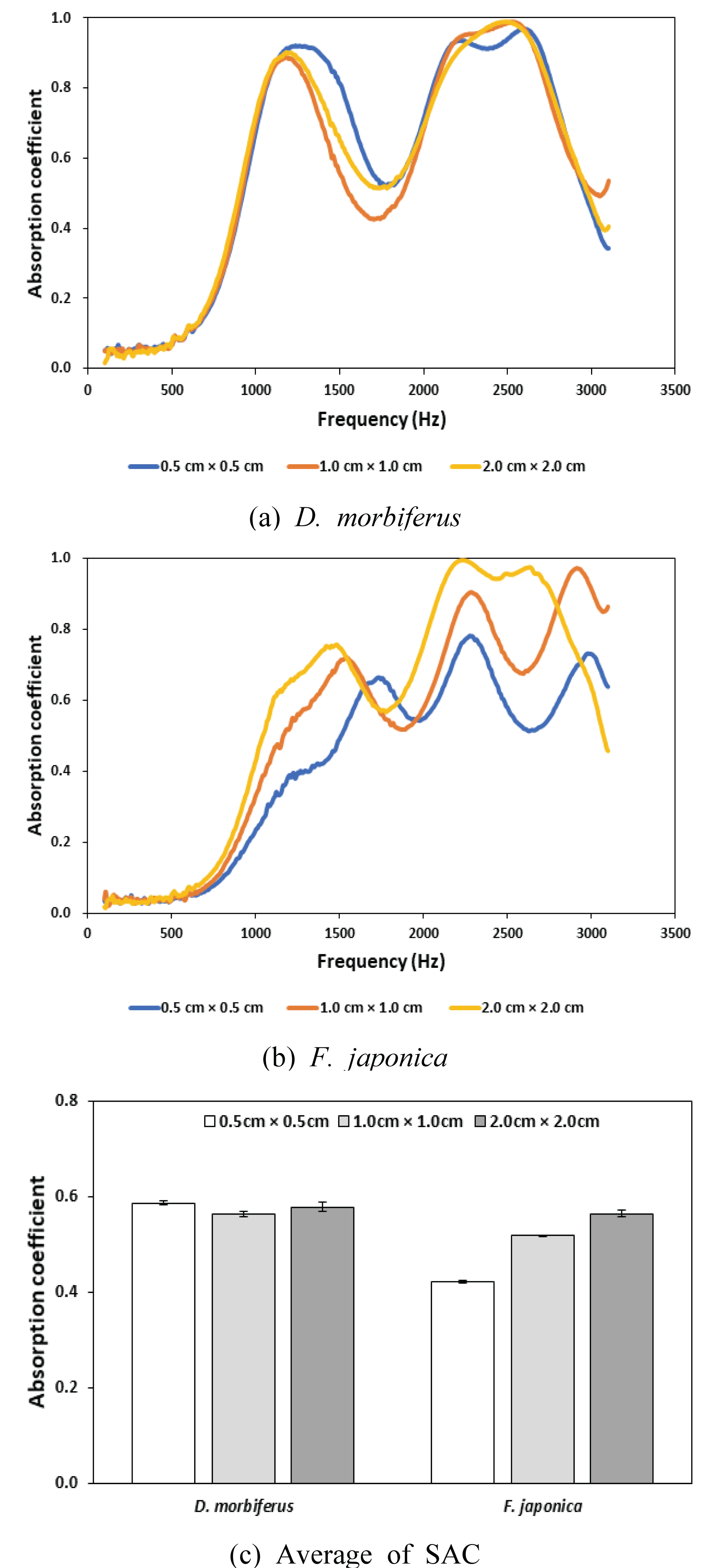
Fig. 5(a)~(b) show the variation of SAC, according to the frequency, of leaves and sponges with the same thickness of 2.50 cm.
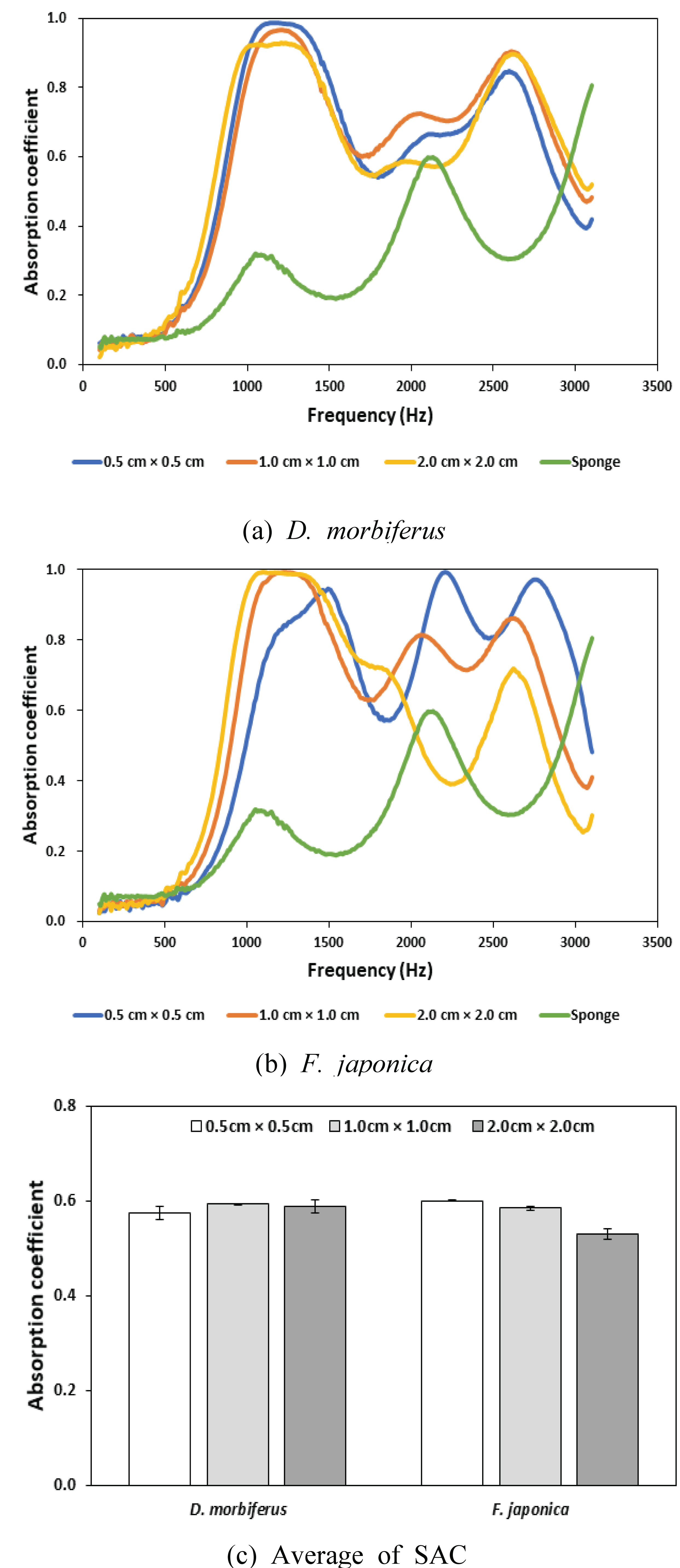
As a result of comparing the variation of the SACs indicated by the leaf size with the increase in the frequency between the two species, D. morbiferus showed more stable results than F. japonica.
In Fig. 3(b) and Fig. 5(b), as the frequency increased, the SAC of leaf size 2.0 × 2.0 cm2 in F. japonica was relatively low compared to other leaf sizes, and these results were different from the 1.00 cm thickness condition. That is, under the 1.0 cm thickness condition, the SAC of leaf size 2.0 × 2.0 cm2 was higher than that of other leaf sizes, especially at the high frequency range of 3,000 Hz. Meanwhile in Fig. 3(c) and Fig. 5(c), it was found that the difference in average of SAC according to the size of the leaf under the condition of 2.50 cm thickness was not significantly higher than that of the 1.00 cm thickness condition.
From the above results, the highest SAC of D. morbiferus under the 2.5 cm leaf thickness condition was found to be a combination of 0.5 × 0.5 cm2 leaf size and frequency range 1,000~1,500 Hz. In the F. japonica species, the coefficient was high in the combination of 0.5 × 0.5 cm2 leaf size and 2,000~2,500 Hz, and 1.0 × 1.0 cm2 size and 1,000 Hz, respectively.
In Table 2, the average SACs at the leaf size of 0.5 × 0.5 cm2 and 1.0 × 1.0 cm2 in the F. japonica species were highest, respectively, 0.601 and 0.585. These results are estimated to be higher than the SAC of 2.0 × 2.0 cm2 in the frequency range of 2,000 Hz or higher (Fig. 5) ; The difference in SAC values according to the leaf size was not significantly below the frequency of 2,000 Hz, but the smaller the leaf size from 2,000 Hz, the higher the sound absorption effect.
In addition, in Table 2, the average SACs tended to increase significantly as the thickness increased under both conditions of 0.5 × 0.5 cm2 and 1.0 × 1.0 cm2 in the F. japonica species (p<0.05). It seems that this resulted from a phenomenon in which the sensitivity of the SAC reacted high from the low frequency band early.
Under the pressure in the same range for each species mentioned above, the higher the thickness of the leaf layer, the higher the SAC in both species. These results are presumed to be due to the occurrence of more resistive layers and air gaps occurring in thicker leaf layer conditions than in thinner leaf layer conditions.
Therefore, the results of the SACs for each frequency band analyzed by the composition of the leaves of the two evergreen broad-leaved tree species performed in this study are considered to be valuable as basic data for use as an environmentally sound insulation material.
4. CONCLUSION
In this study, the SAC of Dendropanax morbiferus and Fatsia japonica as subtropical evergreen broadleaf tree species were estimated and compared sound absorption effects of each specimen; the leaf layer thicknesses of 1.00 cm, 1.75 cm, and 2.50 cm and the leaf sizes of 0.5 × 0.5 cm2, 1.0 × 1.0 cm2, and 2.0 × 2.0 cm2. The measured values of sound absorption effects for all conditions were statistically analyzed by Duncan's multiple range test. The SAC at thickness of 1.00 cm and 1.75 cm for both evergreen broadleaved tree species was generally increased with the leaf size, respectively. The SACs of 1.75 cm thickness were mostly greater than that of 1.00 cm thickness in each leaf size for both tree species. However, as the leaf layer thickness increased, the difference in sound absorption effect according to leaf size tended to decrease. There was no significant difference in SACs according to leaf size of D. morbiferus at 2.5 cm thickness condition, and there was a significant difference in F. japonica, but it was significantly weaker than that at 1.00 cm thickness condition. The 2.50 cm thick leaf layer composed of leaf specimen size 0.5 × 0.5 cm2 of F. japonica has the highest sound absorption effects for all conditions in this study and is expected to be used as a material for natural sound absorbers.