1. INTRODUCTION
Wood, as a natural material, has various excellent properties and has been extensively utilized over centuries. However, wood is easily subjected to biodeterioration caused by microorganisms (Cao and Yu, 2010; Lee et al., 2017; Won et al., 2016; Won et al., 2017), which greatly limits its utilization. An effective way to provide wood with decay resistance in practice is by treating with preservative (Humar and Lesar, 2008; Kartal et al., 2015; Lee et al., 2016; Sen et al., 2009). As a widely used water-borne preservative with outstanding properties, Copper Azole (CA) is not waterproof (Tascioglu and Tsunoda, 2010; Tang et al., 2013). As a result, cracking and warping of CA treated wood always take place, and thus the leaching of preservative occurs. Therefore, a compound treatment on wood with both preservative and water repellent is an efficient consideration to enhance the dimensional stability of water-borne preservative treated wood. There has been several reports concerning this. Specifically, in the 1990s, foreign researchers started to add water repellent into preservative to impregnate wood (Yang et al., 2016). More recently, the wood preservative industry in China tried to combine waterproofing agent with preservative to modify wood for outdoor applications (Chen et al., 2009). Wang et al. (2011) treated Pinus massoniana Lamb. with waterproofing agent and preservatives simultaneously and found that water repellency and wet-resistant rates of modified wood were greatly improved. Besides, Lesar et al. (2011) pointed out that wood impregnated with both water repellent (wax emulsion) and preservatives (boron compounds) was protected against decay effectively.
In our previous research, environmentally friendly paraffin water repellent was prepared based on natural surfactants of alkyl glycoside and span (Wang et al., 2018). It was proved that wood treated by this repellent with CA had a good performance of water resistance and dimensional stability (Wang et al., 2014), metal corrosion resistance (Wang et al., 2015), anti-mould and anti-blue stain (Zhong et al., 2014) and decay resistance (Liu et al., 2018). Interactions among the waterproofing agent, preservative, and wood were further investigated by stress relaxation approach (Liao et al., 2016). However, the leachability and its effect on decay process of treated wood have not been characterized yet.
Generally, the decay resistance is mainly evaluated by the mass loss of wood. Namely, the amount of wood components including cellulose, hemicellulose, and lignin will be reduced due to fungal infection (Bari et al., 2015; Liu et al., 2018). However, this traditional method only reflects the change of quality before and after wood decay rather than microscopic alterations in wood. Fourier transform infrared spectroscopy (FTIR), a favorable methodology for analyzing functional groups and chemical structures of materials, is fast and highly sensitive, which has been widely applied in the researches of wood science including the early diagnosis of wood biodeterioration (Wang et al., 2009). For instance, Li et al. (2010) studied the chemical changes of masson pine (Pinus masso-niana lamb.) infected by brown-rot fungi with FTIR, and confirmed that FTIR could be used to accurately investigate the content of holocellulose and Klason lignin during the decay process. Fackler et al. (2011) provided evidence for white-rot decay process in wood cell walls using FTIR microscopy. However, the destruction of cellulose could not be fully understood by FTIR. X-ray diffraction technique (XRD), being capable of detecting the crystalline structure in cellulose, can be used to analyze the variation of cellulose crystallinity. Therefore, it is an effective way to follow the changes of the main components in wood cell walls, and therefore the functions of the compound system against decay during biodegradation process by combining FTIR with XRD.
The objective of this work was to characterize the laboratory decay resistance of poplar wood treated with the compound system of paraffin emulsion and CA. The influence of leachability on the decay resistance against white rot fungi of the treated wood was evaluated by the mass loss. To interpret the decay mechanism and effects of the compound system, especially for the early stage, FTIR and XRD were employed to obtain time-dependent changes of functional groups and crystallinity of chemical components in wood cell walls during the decay process. This research was undertaken to provide the scientific basis and techniques required support for the development of waterproofing preservatives, which is of great importance to achieve high efficiency and high value-added utilization of fast growing wood.
2. MATERIALS and METHODS
Poplar (Populus cathayana), a fast growing wood in north China, was chosen as the test species. The sapwood, free of obvious defects (decay and knot), was used and cut into samples with the size of 20 mm (T) × 20 mm (R) × 10 mm (L). The density was 0.38 g/cm3, with moisture content of 8-12% in an air-dried state.
Wood preservative was CA, purchased from Guangzhou Xingyue Wood Preservative Limited Company in China. The main components were copper and triazole at 9.98% and 0.42%, with active ingredient content being 10.4% in total. Use category of C3 (outdoor without soil contact) and C4A (outdoor with soil contact) were applied in this study. According to the American Wood Protection Association Standards (AWPA U1-08, 2008), the minimal retention levels of CA for the two conditions used were 1.7 kg/m3 and 3.3 kg/m3 respectively, corresponding to concentrations of 0.3% and 0.5% determined by a pretest, labeled as 0.3%C and 0.5%C.
Paraffin wax emulsions, as the water repellent, were prepared in the laboratory based on natural surfactants of alkyl glycoside and span at solid content of 25% (Wang et al., 2018). Two concentrations of paraffin wax emulsion selected in this work were 0.5% and 2.0%, labeled as 0.5%W and 2.0%W. This resulted in four uniform and stable compound systems prepared by physically mixing CA, paraffin wax and deionized water, labeled as 0.5%W/0.3%C, 0.5%W/0.5%C, 2.0%W/0.3%C, 2.0%W/0.5%C shown in Table 1.
Coriolus versicolor (L.) Murrill, as the typical white-rot fungi in China, chosen as the experimental fungus in this work was purchased from the Chinese Academy of Forestry.
The samples were first oven-dried at 103 °C to constant mass W1 (g). Then a full-cell process was applied one-time to treat the samples by CA, paraffin emulsion, and their compound systems. Specifically, they were exposed to a vacuum condition of -0.1 MPa for 30 minutes, followed by a pressure of 2 MPa for 1 h. After impregnation, the samples were taken out, and liquid on the surface was wiped away by blotting paper. The treated samples were kept for two days at room temperature. Finally they were oven-dried at 103 °C to constant mass W2 (g). Weight percent gain (WPG) of the treated wood was calculated by Equation (1).
Leachability of CA as well as the compound system treated wood was determined according to the standard of E11-06 issued by AWPA (AWPA E11-06, 2006). Specifically, the samples were first immersed in 300 ml deionized water in a beaker and agitated with a magnetic stirrer bar at 3000 rmp/min. The leachate was removed and replaced after 6, 24, 48 h and thereafter every 48 hours with the same amount of deionized water. The samples were leached in total for 14 days and their content of Cu before and after the leaching was measured by ICP-AES (Perkin 5300V, USA) after acid digestion according to AWPA standard A21-14 to determine percentage copper release (AWPA A21-14, 2014) The Cu leaching rate (LR) was calculated by Equation (2).
Where L1 and L2 represent cooper content before and after leaching respectively.
Laboratory decay resistance test was conducted according to China Standard GB/T 13942.1-2009. A 300 ml cylindrical culture bottle with a plastic screw lid was used as the decay chamber, containing the following ingredients uniformly mixed as: 150 g of sand, 75 g of wood powder, 4.3 g of corn flour, and 0.5 g of brown sugar. Two feeder strips of poplar with the size of 25 mm×25 mm×3 mm were placed on the top of the mixture, and 8% malt solution was added into the bottle which was autoclaved for 60 min at 121 °C for sterilization. White-rot fungi was inoculated on the edge of the feeder strip in the medium under aseptic conditions. The entire mixture was kept at constant temperature (25±2 °C) and humidity (65%-75%) for 2 weeks. When the hyphae grew to cover the two feeder strips, test samples were autoclaved for 30 min at 103 °C and placed in the medium for 12 weeks. Each bottle had two replicates that were on the top of the strips. As shown in Table 1, all the nine groups of wood samples were tested for decay resistance and six replicates were used in each group. In addition, three of the groups (Control, 0.5%C, 2.0%W/0.5%C) were added six more blocks for decay process characterization. According to China Standard GB/T 13942.1-2009, wood decay resistance is divided into four grades based on the mass loss as shown in Table 2.
Wood decay resistance grade | Mass loss | |
---|---|---|
I | Very durable | 0~10% |
II | Durable | 11%~24% |
III | Slightly durable | 25%~44% |
IV | Non durable | >45% |
The samples were crushed into 60-80 mesh powders and oven-dried at 103 °C. The powders were mixed with potassium bromide (KBr) which was used to correct the background and oven-dried at 120 °C as well, at a ratio of 1:10. The mixture was pressed into thin slices with a pressure of 4 MPa and monitored by FTIR (Bruker Vertex 70v, Germany). The scanning range was 1000-3500 cm-1 with a resolution of 4 cm-1, and the scanning number was 32 times.
60-80 mesh sample powders were oven-dried at 103 °C and characterized by XRD (Bruker D8 ADVANCE, Germany) at scanning angle of 5°-40° (2θ) and scanning speed of 4 °/min. Crystallinity of the samples was acquired by using Segal empirical formula (Li et al., 2017).
3. RESULTS and DISCUSSION
Weight percent gain and mass loss after decay resistance testing for the treated samples are listed in Table 3. It is clear that the weight percent gain increases with the increase of treating concentration.
As shown in Table 3, the Control exhibited the maximum mass loss of 64.4% (p<0.05), followed by paraffin wax emulsion treated wood, both corresponding to durability class of “non durable”. The results support the report by Liu et al. (2018). Fortunately, after CA or compound system impregnation, the durability class of treated samples became “very durable”, proving CA and its treated wood had excellent performances after leaching. Compared with CA treated wood, the mass losses of compound system treated wood at 0.5% paraffin wax emulsion were lower (p>0.05), while the opposite result was obtained for the 2.0% paraffin wax emulsion (p>0.05), indicating that paraffin water repellent at high concentration may have a negative effect on the impregnation of CA for the compound system treated wood (Wang et al., 2018; Liu et al., 2018).
As shown in Fig. 1, the Cu leaching rate of high concentration CA treated wood (0.5%C) was more than twice as much as that of low concentration (0.3%C). The Cu leaching rates increased with an increase in the concentration of CA, which is consistent with the result of Lin et al. (2009).
The reason being that the fixation rate of CA is greatly affected by its concentration. Specifically, the fixation rate of cooper in preservative with high CA concentration is lower than that with low CA concentration (Wang et al., 2013; Jiang and Zhang, 2008). As the fixation rate increases, the Cu leaching rate decreases. The Cu leaching rates of compound system treated wood with the concentration of 0.5% paraffin (0.5%W/0.3%C, 0.5%W/0.5%C) were lower than both corresponding CA treated wood, while those of compound system treated wood with the concentration of 2.0% paraffin (2.0%W/0.3%C, 2.0%W/0.5%C) gave the opposite trend, which suggests that the addition of water repellent at 0.5% concentration level plays a positive role in waterproofing during the process of leaching, whereas in case of compound system treated wood at higher concentration of water repellent, the paraffin emulsion was impregnated into wood to form a layer of wax outside the cell wall (Wang et al., 2015; Liu et al., 2018), hindering CA entering wood cell wall, which leads to a significant reduction in fixation rate of CA. This result of Cu leaching rate for compound system treated wood at two kinds of paraffin emulsion concentrations is consistent with that of mass losses.
The FTIR spectrum is usually divided into three bands, 3500-2700 cm-1, 2700-1800 cm-1, and 1800-1000 cm-1 where main functional groups from holocellulose and lignin of wood are involved (Huang et al., 2015; Wang et al., 2014; Chi, 2005). The spectra for the samples of control, 0.5%C and 2.0%W/0.5%C after decay by white-rot fungi are shown in Fig. 2 and Fig. 4.
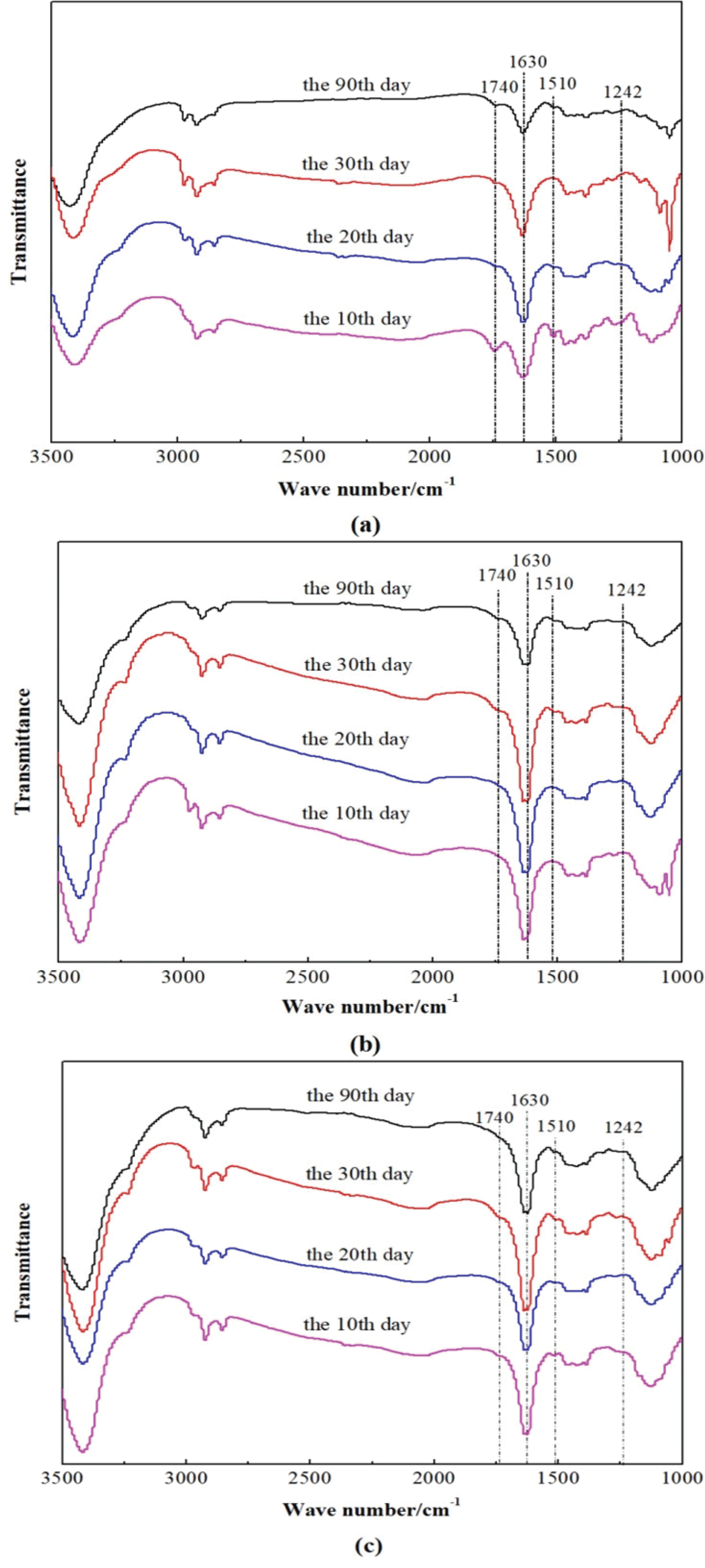
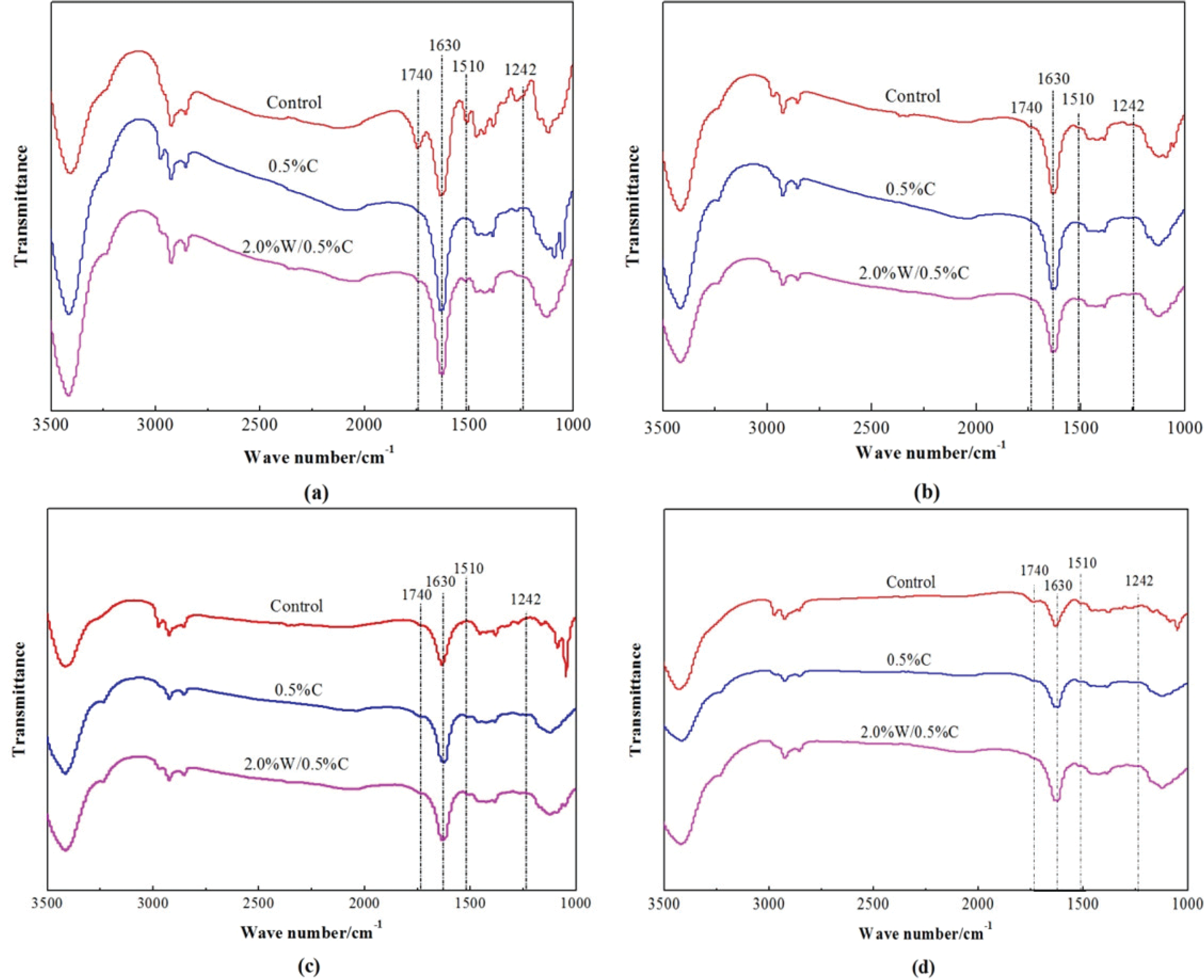
The different characteristic peaks represent functional groups of hemicellulose and lignin, i.e., the peak of 1740 cm-1 attributes to non-conjugated C=O stretching vibration absorption peak of hemicellulose, 1630 cm-1 and 1510 cm-1 corresponds to conjugate carbonyl C=O stretching vibration and carbon skeleton vibration from the aromatic ring of lignin, and 1242 cm-1 belongs to the peak of lignin guaiac. As shown in Fig. 2a, b and c, the peak intensities of 1740 cm-1, 1510 cm-1, and 1242 cm-1 decreased during the decay process, which indicates the chemical components of hemicellulose and lignin were degraded, which is in favor of Pandey and Pitman (2003), Pandey and Nagveni (2007) and Liu et al. (2018). Therefore, the weight of samples after decay dropped as suggested by mass losses.
The intensities of 1740 cm-1 and 1242 cm-1 which were transferred from the absorbance of infrared scanning are shown in Fig. 3. The maximum intensity was taken as 1 and other values are the ratio of the maximum. In Fig. 3a, the intensity of 1740 cm-1 peak of the Control decreased significantly from the 10th to 30th day, indicating that the content of carbonyl was reduced, and xylan in hemicellulose was partly degraded (Lin et al., 2010; Pandey and Pitman, 2010). This peak intensity also decreased from the 30th to 90th day, showing that hemicellulose decomposed not only in early and middle stage, but also in late stage during the decay process. Compared with the Control, the peak intensity of CA and compound system treated wood decrease slightly in both early stage and middle stage, illustrating that CA could reduce the decomposition speed of hemicellulose. At the same time, the intensity of the Control during the late stage was much lower than that of CA and compound system treated wood, illustrating that CA could reduce the degradation amount of hemicelluloses. Besides, it is found that the decay resistance effect of compound system treated wood is not better than that of CA treated wood, suggesting the higher mass loss of the Control could be partly caused by the degradation of hemicellulose. According to Wang et al. (2006), hemicellulose breaks down into small molecular acids (such as glucose acid, lactic acid, etc.) or lipids (glucose esters), which are directly used by the white-rot fungi during the process of degradation.
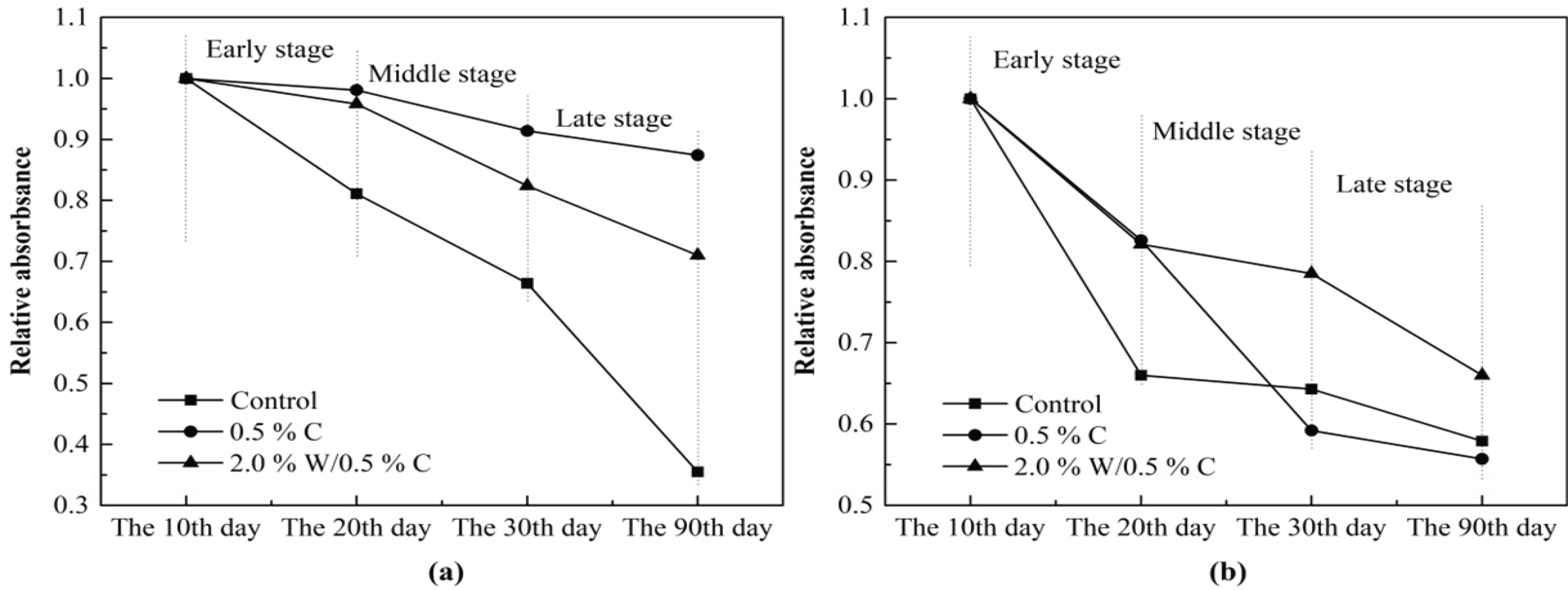
The variety of 1242 cm-1 during decay process is presented in Fig. 3b. The intensity of guaiacyl lignin of the Control was clearly reduced in the early stage, and decreased slightly from the 20th day to the 90th day, which indicates that lignin was degraded extensively in early stages of decay. The long chain hydrocarbon of lignocellulosics was cut into small molecules, and the ring structure was gradually decomposed into chain compounds (Wang et al., 2006). The degradation degree of CA treated wood and compound system treated wood is lighter than the Control in early stage. Therefore, CA could slower the degradation of lignin. The intensity of lignin guaiac of compound system treated wood decreases clearly in early stage compared with the middle and late stage, in accordance with the Control, but the intensity on the 90th day is higher than that of the Control. On the other hand, the final degradation amount of lignin of CA treated wood is much more than the Control.
The FTIR spectra of samples on the 10th day during the decay process is shown in Fig. 4a. The peak intensity of 1740 cm-1 of CA treated wood is much lower than that of the Control. The reason is that the ligand exchange effect between CA and hemicellulose results in an increase of carboxylate anion. In this reaction, a ligand from copper ammonia complex was replaced by the samples component of carboxyl and carbonyl, carboxyl on hemicellulose was split to form carboxylic acid salt, and then form Cu-carboxylate complex with the Cu element. Meanwhile, the esters in lignin could hydrolyze into aromatic carboxylic acid groups under the weak alkaline conditions. (The pH value of CuAz is 8-9), and then react with copper to produce copper carboxylic acid, which also led to the reduction of the absorption peak in 1740 cm-1 (Qin, 2004). The reason why peak intensities of 1630 cm-1, 1510 cm-1, and 1242 cm-1 of CA and compound system treated wood are weaker than the Control is that the phenolic hydroxyl group in lignin is the main fixation site of copper, and after the treatment by copper azole, the H atom of phenolic hydroxyl was replaced by Cu atom, forming a complex of “N-Cu-O”. Meanwhile, the aromatic ester of lignin could hydrolyze into aromatic carboxylic acid group under weak alkaline conditions, and then react with copper to produce copper carboxylate (Wang et al., 2009), which reduced the intensity of absorption peak of lignin.
As shown in Fig. 4b, the peak intensities of 1740 cm-1, 1630 cm-1, 1510 cm-1, and 1242 cm-1 on the 20th day of CA treated wood are higher than that of compound system treated wood and the Control, indicating that hemicellulose and lignin of CA treated wood were only degraded slightly, while those of the Control were severely destroyed.
The FTIR spectra of samples on the 30th day during the decay process is displayed in Fig. 4c. The peak intensities of 1740 cm-1, 1510 cm-1, and 1242 cm-1 of CA treated wood and compound system treated wood are lower than the Control, because CA treated wood is severely degraded in middle decay stage, while the Control is badly decomposed during the early stage, which provides make a further indication that CA slows the degradation of hemicellulose and lignin.
As presented in Fig. 5, the crystallinity of the Control on the 10th day is lower than that of the 20th day, because hemicellulose and lignin in the amorphous region were clearly degraded in the early stage of decay, causing a rise in crystallinity. Meanwhile, it was found that the crystallinity of 20-90 days decreased gradually, which suggests partial cellulose degradation, consistent with Xu et al. (2013) and Liu et al. (2018), namely white-rot fungi degraded hemicellulose and lignin first, and followed by the matrix and cellulose at the same time. Du (2004) pointed out that hemicellulose was degraded first to provide the carbon source and energy for fungal growth, and in the subsequent degradation, hemicellulose and lignin were degraded at the same time, causing degradation and destruction of the compact outer layers structure of the cell wall to further degrade hemicellulose, cellulose and lignin at the same time, and the whole degradation process was an oxidative cracking process. However, the analyses could not determine whether cellulose is degraded from 0 to the 20th day.
The crystallinities of CA and compound system treated wood (0.5%C, 2.0%W/0.5%C) vary slightly during the 10th day to 30th day, but increased on the 90th day, demonstrating that hemicellulose and lignin were considerably degraded. Moreover, CA has a protective effect on cellulose. The reason is that a part of copper was absorbed by cellulose, and there is a physical adsorption effect between copper and cellulose, such as the Van der Waals force (Wang, 2007). The crystallinities of samples after decay agree with the results of mass losses. The mass losses decreased with an increase of crystallinity.
4. CONCLUSION
In this work, the sapwood of Populus cathay was treated by CA, paraffin water repellent, and compound systems to study in the laboratory decay resistance, with a consideration on the impact of leachability, and the decay process was investigated applying the methods of FTIR and XRD. The decay resistance levels of compound system treated wood after leaching were “very durable”. Hemicellulose was degraded during the whole decay process, while a large amount of lignin was decomposed at early stage as suggested by FTIR analysis. Combined with XRD, it was found that the white-rot fungus C. versicolor degraded hemicellulose and lignin during early decay, and then decomposed hemicellulose, lignin and cellulose in middle and late stages. Moreover, CA could reduce the degradation speed of hemicellulose and lignin, and decrease the degradation amount of hemicellulose. These findings could provide practical references and theoretical understanding for protection of wood against decay.